Hold It: Energy Storage
Have you heard of Sisyphus, from Greek mythology? After committing some heinous crimes against the gods (cheating chief death-dude Hades twice), he was given the bizarre punishment of repeatedly pushing a boulder up a hill, only to watch it roll right back down. Then repeat the process for the rest of time. Sisyphean labor is pointless, unrewarding, and soul-sucking.
What if Sisyphus’ labor, though, was actually productive, unbeknownst to him? As he pushes his boulder up the hill, it gains potential energy. Once he lets go of the rock, potential energy transfers into kinetic energy, a type of energy that can be converted to electricity in various ways. If Sis could time the rolling to when electricity is needed, it could be put to use, to jam out some electric lyre music, or however an ancient Greek might use power.
Sisyphus’ is a relative of Aeolus, the keeper of winds in Greek mythology, so would know full well that wind doesn’t always blow. Today’s taller wind turbines have average capacity factors around 40% onshore and 50% offshore; solar tops out at half this much. Long-distance transmission can help with intermittency quite a bit, but the ability to store energy on a more local basis is also quite desirable. We’ll see that some of the least intrusive energy storage methods seem quite Sisyphean in their design, but might end up being quite important in allowing wind and solar to provide the majority of electricity needs.
Microgrids
Power generation doesn’t just have to be balanced on average, it has to be matched with demand moment by moment. Each time air conditioners turn on across a city, or electric toasters start cooking breakfast, the generation from power plants must also be ramped up an equivalent amount. If generation doesn’t match the load, then the frequency of the alternating current changes, harming infrastructure and appliances. In extreme cases, a blackout can occur, which can be a huge challenge to get back online.
Since load has to be matched at all times, it takes some preparation for a small system to be able perform this balancing. A house with solar panels can provide its own power in a blackout only if storage is available. When a rechargeable battery is added to a solar array, then the house can provide its own power at all times (until the batteries run out). These systems that can work completely on their own are called microgrids.
Microgrids can be designed on a larger scale than a single house: a college campus, neighborhood, or entire city, as long as there’s storage that can be applied at any time. The benefits of such a system are huge, especially when one considers the increased severe weather that we’re facing in the future. Microgrids can start back up quickly, even when power lines are down.
Power demand cycles in the US
With solar panels becoming so cheap and with the large benefits of local power production, let’s consider what kind of storage is needed to balance the load for a microgrid. Let’s consider a neighborhood scale, with some residential, commercial and industrial loads. We’ll use examples from the daily cycle of generation and load across the world, starting with the United States.
Loads in the US have a pronounced daily cycle, with much more power required in the day than at night. Peak hours are typically defined as 7 AM to 11 PM local time. This is good for solar generation; the sun is up most of the time when we need power. Loads also tend to be higher in summer than winter, largely due to the need for air conditioning. This is also in sync with solar generation potential, since the Sun is higher in the sky and daylight hours are longer in the summer.
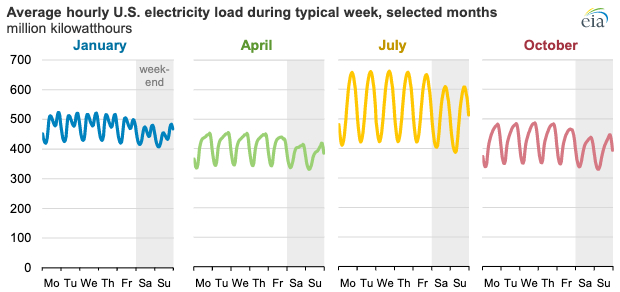
The biggest asynchronicity between loads and solar power potential tends to be in the evening. Loads remain high when people come home from work, turn on air conditioning or appliances. The need for evening power means that some load balancing problems with solar can be dealt with by having just a few hours of storage. California, a state with large nameplate capacity for solar, is a particularly interesting case to examine on a day to day basis.
In large countries, the variation of sunset across the country can help to even out the loads. For instance, on the east coast of the US, evening peak loads can occur while the Sun is still up in the Mountain West. Adequate transmission over long distances can help to share daylight across the world.
Types of storage
BATTERIES
Lithium ion batteries, despite their environmental impact, are becoming much cheaper, and even though their original design was for usage in vehicles, they are being adopted in other applications, including back-up power storage for houses or entire power grids. Repurposed batteries from electric vehicles are starting to be used as backup storage. An aged battery might not have the desired performance needed for long-distance driving, but can still be quite useful for stationary sources. It’s important to note that the largest electric car batteries of today (around 100 kWh) are often quite sufficient as backup for entire buildings, even when depleted. Typical large home backup systems are roughly 10-20 kWh, 5-10 times smaller than the storage that zips along with drivers down highways in long-range electric vehicles.
Utility-scale battery storage can reach into the million kWh (GWh) range. Many of this storage provides around 4 hours of power at its maximum rate of discharge. In addition to their energy storage capacity, other important numbers for energy storage are how much power they can provide, and whether it takes time to ramp up to the maximum power delivery. Batteries can deliver their maximum power within a fraction of a second. Molten salt storage, which is used in some concentrating solar power plants and proposed for new nuclear plants, can be ramped up within around one hour.
Batteries for grid storage don’t have the space or weight constraints like electric vehicle batteries do, so different chemistries can be appropriate. Saltwater batteries use saline solutions as the electrolyte, meaning no toxic chemicals are involved. Unlike lithium ion batteries, saltwater batteries are non-flammable, and can be run for many more charge cycles, around 10,000 compared with 1,000 for Li-ion. They are also low cost and easily recycled. On the down side, saltwater batteries require twice the space as other chemistries and discharge more slowly, meaning less power delivery.
Flow batteries are a technology that’s used as a backup at some power plants. Vanadium redox flow batteries last a very long time, and can provide high power. It’s simple to control power and energy separately with these batteries. Adding more tanks gives more energy storage capacity. On the downside, vanadium is a quite dangerous material. There is active research on many other battery chemistries at universities, government labs and companies around the world.
PUMPED HYDROELECTRIC
Worldwide, one type of energy storage is used vastly more than all the rest: pumped hydroelectric, which makes up a full 95% of the current utility-scale storage worldwide. Pumped hydro works like traditional hydroelectricity when generating electricity, by turning a turbine from water that’s flowing downhill due to gravity. In times of excess electricity generation from other sources, storage of energy is created by pumping water uphill into some kind of reservoir.
Water is a high density substance, meaning hoisting it upwards requires a lot of energy. Potential energy is mass times gravity times height. A cubic meter of water (1000 L), which weighs exactly 1 ton, therefore stores 9,800 J (2.7 Wh) for each meter it is lifted. If pumped upward 100 meters, 3.67 m3 of water is required for every kilowatt-hour of storage. Again for a 100 meters of upward pumping, a hectare of reservoir size that is one meter deep stores 2.7 MWh of gravitational potential energy.
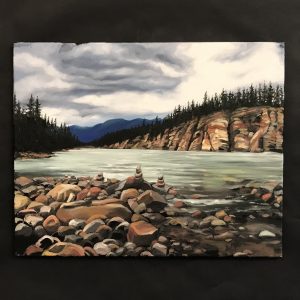
The upper reservoir in a pumped hydro facility can be either a natural lake or a human-made reservoir. The lower reservoir can be a human-made reservoir, a river, a lake or even the ocean. When both reservoirs are human-made, the system is called “closed loop,” and it’s thought that such systems are less damaging to the environment. All pumped hydro storage in the US are open-loop systems, with one end connecting to a natural water source.
Pumped hydro storage averages around 80% efficiency, meaning not much energy is lost in the process. Like hydroelectricity, it can ramp up or down very quickly, so pumped hydroelectric energy storage can be used to provide peaking or load following power.
The world storage capacity for pumped hydroelectricity is 1.6 TWh, including 0.25 TWh in the United States. The nameplate capacity of this storage (the maximum electricity that can be provided if all the turbines were turning at once, discharging their energy) is 181 GW, with 29 GW in the US. There are many more sites than are used: it has been estimated that 22,000 TWh are available worldwide, which is almost 2 TW-years!
However, pumped hydroelectricity is associated with many of the same environmental concerns as traditional hydroelectricity. The creation of reservoirs often requires flooding of land, and disruption of ecosystems. Closed-loop systems are less likely to have negative impacts on surface and ground water quality. Previous industrial sites like mines could potentially be used as a reservoir, although these might be more likely to pollute groundwater. Water from closed-loop systems are more likely to need replenishment to offset evaporation as well, potentially from groundwater.
GRAVITATIONAL STORAGE
Hold up! Water is not the only substance that can be lifted. Gravitational energy storage can also be designed to lift solid objects upward and downward to store and release energy. The use of solid materials can give a mass benefit, since they can be more dense than water. A cubic meter of steel weighs around 8 tons, while concrete and fiberglass weigh around 2.5 tons/m3, and most rocks are between 2 and 5 tons/m3, compared with water’s 1 ton/m3. The hope would be that the carbon intensive materials like steel and concrete would not have to be created for use in an energy storage system, and some materials that would otherwise go to landfills could be utilized.
The sheer amount of mass needed is a little daunting. An entire city block length’s worth of cars (36.7 tons worth) would have to be lifted 10 meters in the air to provide just a single kilowatt-hour of energy storage. That’s only long enough to extend the typical household’s energy usage one hour. On the other hand, gravitational storage doesn’t degrade like battery chemistry, and could potentially be ramped up quickly to meet demand.
Several types of gravitational storage systems have been designed, and are beginning to be tested around the world. An initial design developed by Energy Vault used hundreds of 35 ton bricks lifted by six cranes, all stacking and unstacking bricks simultaneously, although they have recently pivoted towards building-like structures. Other companies are planning to experiment with underground storage, utilizing abandoned kilometer-deep mineshafts. Others prefer keep their weights entirely at ground level, using train cars that move mass up and downhill. There’s a project being built in Nevada that will move a fully loaded train upwards along a short track to store up to 12.5 MWh of energy. It should produce up to 50 MW of power for 15 minutes, using 210 train cars on 10 tracks, weighing a total of 68,000 tons, around 320 tons per car. Gravitational energy storage is heavy stuff!
FLYWHEEL STORAGE
Flywheel storage uses fast-spinning wheels to store and release energy. These systems are often made with steel or carbon fiber, are highly durable, and can run in a vacuum or with magnets to reduce friction. They ramp up incredibly quickly, within milliseconds, meaning they can help with improving the quality of electricity in a microgrid. Flywheels can also discharge their stored energy rapidly.
Flywheel energy storage is often used for charging up cars or buses, because they can rapidly recharge a vehicle battery, or in industrial applications where large amounts of power are needed for a short duration. Another advantage of flywheel storage is that the amount of energy stored at any time can be calculated easily, which is surprisingly difficult to do for electrochemical battery charge. A disadvantage of flywheel storage is that they lose energy relatively rapidly, often around 3% per hour. This means they are only good for relatively short time period storage, which is just fine for charging up a bus that goes out on a couple hour long route, but needs to be charged as quickly as possible when it’s back at the depot. Flywheels have great benefit over batteries in terms of environmental impact, and have a potentially bright future in providing storage and improving power quality within microgrids, even at the house or neighborhood scale.
COMPRESSED AIR STORAGE
It might seem that a substance as light as air could never compete with water for storing energy. But gases can be compressed, and large underground formations exist that can hold tremendous amounts of air under high pressure. Compressed air can be used for energy storage in several ways, and a few such plants exist around the world.
Conventional compressed air storage involves augmenting fossil gas burning, so we won’t discuss that option. Adiabatic compressed air energy storage (ACAES), on the other hand, involves no fossil fuels. ACAES plants work by compressing air underground when there’s excess electricity, and unleashing blasts of the high-pressure gas to turn a turbine when power is needed. An ACAES facility recently came online in Ontario, a small demonstration project with 10 MWh of storage capacity and 1.75 MW power delivery. But follow-on projects that are being developed are 500 times this size, including two plants in California in planning with around 5 GWh of energy storage capacity. This would be larger than any non-pumped hydro storage anywhere around the world.
Time will tell whether any of these Sisyphean structures will catch on. Perhaps instead of Sisyphus’ punishment, these repetitive movements should be thought more through the lens of Terpsichore, the ancient Greek muse of dancing, directing complex rhythms that smooth out the chaotic pulses of wind and sunshine.
Transmission
When you turn on your device to read another chapter of Climate, Justice and Energy Solutions, it’s possible that those electrons were set in motion by a process that started quite a long distance away, involving several different transformations of the electric current. Let’s say your power is generated by offshore wind turbines, and needs to be transmitted more than 1000 km to your location. It’s ideal to have efficient methods of increasing voltage, so that transmission of electricity can occur with lower resistance, and then decreasing voltage, so that around neighborhoods and residences the power lines are safe. Transformers more than meet the ideals, increasing voltage near power plants in step-up substations, and then back downwards in neighborhood step-down substations near the loads. Transformers on poles provide one final step-down of voltage before power enters residences.
Countries like China have been investing large amounts of resources into high voltage direct current (HVDC) power lines, which are the most efficient for long distance transmission. It is possible to convert existing transmission lines, or simply build new lines. In the US, the lack of a national grid and unwillingness to make large public investments in transmission have been major impediments. Better transmission would allow for incorporation of more renewables into the grid, for instance, bringing wind power on agricultural land into cities. A good transmission system adds resiliency as we face increasing weather disasters, and can allow for less storage needs as well.
Connect
The Sunrise Movement is a youth climate justice organization founded in 2017. In addition to work at the national level, they have over 400 hubs all across the United States, in cities and on college campuses. They work to connect climate justice with racial and economic justice, and have helped launch the Green New Deal into national prominence.
- Check out the Sunrise Movement’s book Winning the Green New Deal, a series of essays by leaders such as Varshini Prakash, Rhianna Gunn-Wright, Bill McKibben, Rev. Dr. William J. Barber II, and Colette Pichon Battle.
- Try out their personality quiz to see what kind of Green New Deal career might be good for you.
An electricity grid that can function on its own, balancing load with generation, even if separated from the larger power grid.
Pumped hydroelectric stores gravitational potential energy by pumping water upward when extra electricity is available. The water can then be used later to turn a turbine to create electricity.
Gravitational energy storage systems store potential energy by lifting objects when electricity is available. The objects are lowered when energy is needed, using the energy to turn a turbine and create electricity.
Flywheel energy storage spins a wheel faster when there's extra power available, and uses this rotational energy to create electricity when needed.