Charging Forward: Lithium-Ion Batteries
Lithium-ion batteries have propelled the electric vehicle industry towards profitability, and more and more batteries are being produced each year. It’s becoming clear that lithium-ion batteries will play an important role in storing energy not just in vehicles but potentially on a global scale, gathering electrons from by solar panels in the daytime, and releasing them to the grid at night. In 2023, installation into EVs alone totaled about 750 GWh, up from 165 GWh in 2020. How do these batteries work, what kind of extraction do they require, and are there other potential technologies?
Batteries store chemical energy that can be converted into electricity, and rechargeable batteries can access the charge and then resupply it when plugged in. Batteries are designed to perform well in the following tasks:
- Storage capacity (must hold lots of charge)
- Speed of energy exchange (must be able to provide lots of power, and recharge quickly)
- Safety (many lithium ion batteries struggle with fire danger)
- Lifetime (should be able to perform many charge-discharge cycles without degradation)
- Small size/weight (much of the mass of an electric vehicle is the battery)
- Good materials (defined by corporations as low price, but material extraction should have humane conditions for workers, and create as little environmental damage as possible).
As element number 3 on the periodic table, lithium is an extremely low density element. It is the only solid element that can float in water, until it reacts with it at least. It is also extremely electropositive, meaning it is easy to get its outer electron moving. When its electron takes off, it leaves behind a positively charged lithium ion. Its lightness and ease of ionization is why lithium works so well for mobile energy storage. Compared with other batteries like lead acid and nickel cadmium, much less toxic materials are used in lithium ion batteries, but this does not imply a lack of environmental damage in mining.
Lithium compounds are used as the cathode, or positive electrode, of lithium ion batteries. Lithium is used along with other elements like nickel, cobalt, lead, aluminum, phosphorous and oxygen to give it stability before being charged up. Charging the battery releases lithium ions, which travel through an electrolyte layer and over to the anode, which is usually made of graphite. The electrolyte serves to allow only lithium ions, and not their recently-departed electrons through that direction. Instead electrons flow all the way through the circuit in charging. Once all lithium ions and their electrons exist within the anode, the battery is fully charged.
The battery releases energy when the lithium ions surge back towards the cathode into their stable compound, while electrons flow through the electric motor and make the car go. Lithium can be used in very thin layers, and don’t require much electrolyte either. After wrapping all this layers of metal like copper to store the charge, the final cell looks a lot like a regular AA battery, although usually a little larger.
Many recent electric vehicle models have battery packs of around 100 kWh. One such pack is made up of 16 modules, each with 516 cells each, for a total of 8,256 cells. If you’re used to changing out AAA batteries around your house, you’ll be pleased to know that it’s not necessary to open up the car to swap in new cells. Because temperature control is a serious problem with these batteries, coolant is often piped through car battery systems for safety and stability.
The 100 kWh battery pack weighs a total of 625 kg, about one third of the total car weight. Batteries this size contain around 10 kg of lithium. The lithium compound used in these packs is lithium-nickel-cobalt-aluminum oxide. Each of these four metals involve troubling mining practices, with cobalt of particular concern, as we’ll detail in the next chapter. It’s not necessary to use cobalt; some lithium ion batteries instead use lithium iron phosphate or lithium manganese oxide. Others are trying to replace cobalt with more nickel. Cobalt helps with stability and lifetime of batteries.
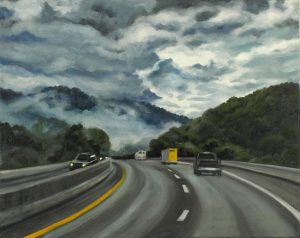
Most e-waste, including lithium ion batteries, goes straight to the landfill or incinerator. Recycling rates are rather small, only around 5% in Europe. It can be dangerous to remove lithium due to fire danger, and accidents have occurred within crushers as well when lithium batteries are put it by mistake.
Lithium rising
Lithium ion batteries have dropped in price tremendously over the last decade. Prices in 2010 were $1100/kWh, but currently are only $115/kWh. Along with the price drop, lithium ion batteries are being used in more and more applications, including many that they were not originally developed for. Sometimes this can be beneficial, like the use of old car batteries to provide backup power for solar arrays. They’re also being used in transportation modes that might replace cars for certain people, like electric bikes. E-bike batteries are typically 0.5 kWh only, so require a fraction of the materials as an electric car.
Even backup batteries for an entire house are significantly less in size than large car batteries of today. The Tesla Powerwall is 13.5 kWh, and other home storage systems are only 6.5 kWh per unit. The smallest new battery electric vehicle, the MINI Cooper SE, has a 32.6 kWh battery, good enough for a 114 mile (183 km) range.
The Ford F-150 Lightning electric pickup truck have batteries sizes of 98 or 131 kWh for standard and the extended range version. The ranges of the standard and extended range F-150 models are 230 and 300 miles (370 and 483 km). Not to be outdone, the Hummer EV comes with a 212 kWh battery.
Let’s put this all in perspective. The battery capacities of these vehicles are tremendous. The Ford F-150 Lightning is marketed as being able to power an entire house for three days, or up to a week with some conservation. 100 kWh is larger than the average monthly electricity bill for the decent living with minimum energy calculation.
Some EV models are now EPA rated as having over a 500 mile (800 km) range, and many new electric vehicles have a range of over 300 miles (480 km). Without a doubt, prior concerns of limited range of electric vehicles are a thing of the past. But is it really necessary to use this much precious material, when the average driving per day is only 30 miles? Car purchases, especially in areas with strong car cultures, tends to be far from the minimum that a given buyer can get by with, and instead is often closer to the maximum a buyer can afford. Perhaps the environmental concerns of today with bring a new era of reduced car ownership and smaller vehicles, but so far the trends have been just the opposite.
Fuel economy and refilling (the electric version)
As mentioned earlier in the book, electric vehicles are significantly more fuel efficient than their gasoline counterparts, due to the significantly reduced heat loss. New electric vehicles range in energy efficiency from around 15-35 kWh/100 km. For heavy drivers, an electric vehicle can potentially double one’s electricity bill.
The time to charge these batteries depends on both the size of the battery and the type of charger. Charging is also non-linear, meaning more power is used at the beginning of the charging than at the end. But it is useful to compare some approximate numbers. A Level 1 charger is just a regular wall unit, and can provide 1.4 kW, meaning each hour can provide 1.4 kWh of charge. Level 2 chargers are significantly faster, but require a special installation with higher voltage. These provide anywhere from 3.4-17.2 kW. Level 3, or fast, chargers can provide 140 kW. Thus in only 30 minutes, one can fill around 100 km of additional range for even the least fuel efficient electric vehicle.
Because charging is faster at the beginning of the cycle, and since fast chargers are still relatively rare, some charging stations require paying by time rather than energy. With these it is recommended to only charge up to 80% in order to save money.
Connect
Pipeline resistance is critical for kicking the petroleum habit. Preventing pipelines is not only protects the water (and thus the life) of the surrounding areas from frequent spills that occur worldwide with astonishing frequency. Pipeline resistance is for us all, from youth who breathe diesel smoke at their school playground to anyone living near tar sand, oil shale or fracking extraction sites.
- Read Indigenous Resistance Against Carbon by the Indigenous Environmental Network and Oil Change International, which highlights pipeline resistance across North America.
- Follow the Tiny House Warriors in their fight against the Transmountain Pipeline. Read a comic book adaptation of their struggle and listen to a 41-track album of music donated as a fundraiser for the group.
- The East Africa Crude Oil Pipeline (EACOP) is a proposed project to deliver oil from Uganda to the coast of Tanzania, and to the rest of the world via ship. Follow the resistance campaigns against it from the #StopEACOP and OilWatch Africa.