3 Protein Targeting, Vesicular Transport
Session Level Objectives (SLOs): after completing the session, students will be able to:
Nearly all proteins have distinctive subcellular (or, for secreted proteins, extracellular) localizations. Many proteins are directed to specific locations by “molecular zip codes” — structures within the protein that contain targeting information. Membrane and secreted proteins are encoded by 30-40% of our genes.
SLO 1. Describe the major organelles of the secretory pathway.
- Secreted proteins, and most integral membrane proteins, are synthesized by ribosomes at the rough endoplasmic reticulum (ER). These proteins can be co-translationally translocated into the ER membrane (for integral membrane proteins like ion channels), or they can be transferred across the ER membrane (for soluble secreted proteins like antibodies).
- At the ER, proteins are folded with the aid of chaperone proteins. In many but not all cases secreted and membrane proteins are glycosylated: complex carbohydrates are covalently attached to the proteins by ER-resident enzymes.
- Following folding, the proteins are packaged into carrier vesicles and transported to the Golgi apparatus. In the Golgi further post-translational modifications are performed and additional quality control checks are made. The proteins enter the Golgi complex at the cis Golgi, traverse the medial Golgi, and end up in the trans Golgi network (TGN).
Fig. 1. General organization of the secretory pathway. Nu, nucleus. ER, endoplasmic reticulum. GC, Golgi complex. TGN, trans-Golgi network. PM, plasma membrane. - The TGN is the Grand Central Station of the secretory pathway: here, proteins are sorted into different carrier vesicles that can go to many different locations in the cell, including endosomes, lysosomes, and secretory vesicles.
- There are two broad categories of secretory vesicles.
- Constitutive secretory vesicles fuse with the plasma membrane “automatically,” by default. This is a “housekeeping” pathway.
- Regulated secretory vesicles fuse with the plasma membrane only in response to a signal. There are many different types of regulated secretory vesicles in different cell types. They may contain hormones (e.g., insulin), neurotransmitters, blood clotting factors, etc. The commonality is that regulated secretory vesicles are held in reserve in the cytoplasm, until a signal indicates that their contents are required at the cell surface.
- Fusion of a secretory vesicle at the plasma membrane is called exocytosis.
SLO 2. Understand the difference between targeting of integral membrane proteins and secretory proteins.
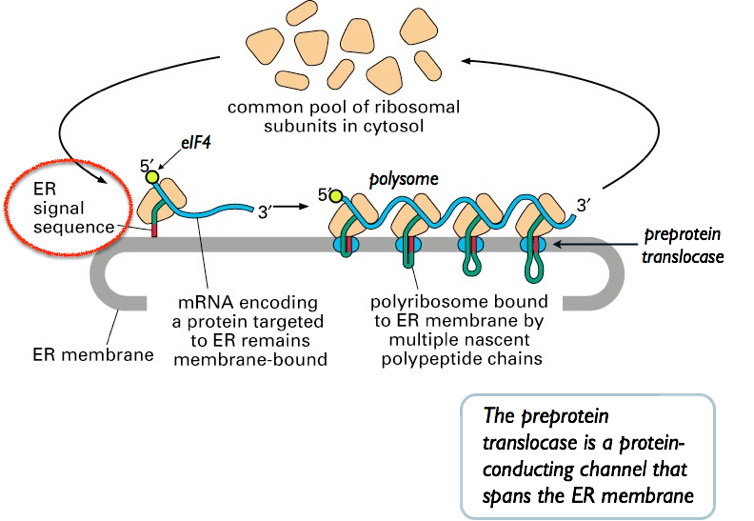
- Integral membrane proteins, including receptors and ion channels, have one or more membrane-spanning domains that traverse the lipid bilayer.
- Secreted proteins generally do not have transmembrane domains, and are soluble in the aqueous lumens of the ER, Golgi, and secretory vesicles.
- Both integral membrane proteins, and secreted proteins, are usually translocated into the ER as they are synthesized. That is to say, translocation is co-translational.
- A common protein machinery is used to target both secreted and integral membrane proteins at the ER: the Preprotein Translocase. This complex is also called the Sec61 complex.
- Recall that the N- terminus is the first part of a protein to emerge from the ribosome. Secreted and integral membrane proteins emerging from the ribosome are marked by a “zip code” — an N- terminal signal sequence
- The signal sequence is identified by the Signal Recognition Particle (SRP). The SRP directs the mRNA, ribosome, and
Fig. 3. SRP recognizes N-terminal signal sequences to target nascent polypeptides to the ER. BiP is a chaperone protein that helps secreted proteins to fold correctly. nascent polypeptide to the Preprotein Translocase.
- As the polypeptide elongates, it is threaded through the preprotein translocase. For secreted proteins, the signal sequence is removed by a site-specific protease. This allows the protein to diffuse in the aqueous phase.
SLO 3. Outline how proteins are folded and modified in the endoplasmic reticulum and Golgi organelles.
- Chaperones in the ER lumen, such as BiP, intercept the nascent protein and attempt to help it fold correctly. In many cases, folding requires the assembly of multi-protein complexes (oligomerization).
- Transmembrane proteins are assembled by allowing trans-membrane segments to escape from the preprotein translocase laterally, into the membrane bilayer (Fig. 4). The transmembrane segments are hydrophobic. Thus integral membrane proteins are soluble (t
Fig. 4. Transmembrane domains are inserted into the ER membrane by lateral escape from the preprotein translocase. hat is, they can diffuse) within the two-dimensional plane of the membrane bilayer, but they are not freely soluble in the aqueous phase.
- 20% of the time or more, the folding of membrane proteins fails! The protein mis-folds: it must then be identified as a “dud” and degraded. The polypeptide is extracted back into the cytoplasm, and degraded by the proteasome (which you will learn about later in the course).
- Mutations, or errors in transcription or translation, can cause mis-folding.
- Chemical and physical stresses (oxidation, elevated temperature, etc.) can cause mis- folding.
- Some amount of mis-folding happens through stochastic processes, even without unusual mutations or stresses.
- Note that quality control occurs both in the cytoplasm and in the secretory system.
- The protein folding and quality control machinery can be overwhelmed. This causes ER stress. The ER stress response is a signaling pathway that does thee major things:
- It senses the amount of unfolded protein in the ER lumen;
- it slows down translation (protein synthesis); and
- it causes transcription of genes encoding ER chaperones.
- Thus the ER stress response is a homeostatic mechanism that allows the efficiency of ER folding to respond appropriately to demand.
- The ER stress pathway can be overwhelmed. At this point, the cell may choose self-destruction (apoptosis).
- Proteins exported to the Golgi are subjected to additional quality control checks for proper folding and post-translational modification. If they fail these checks, they are often retrogradely transported back to the ER. At the ER they get an additional chance to fold or to be degraded.
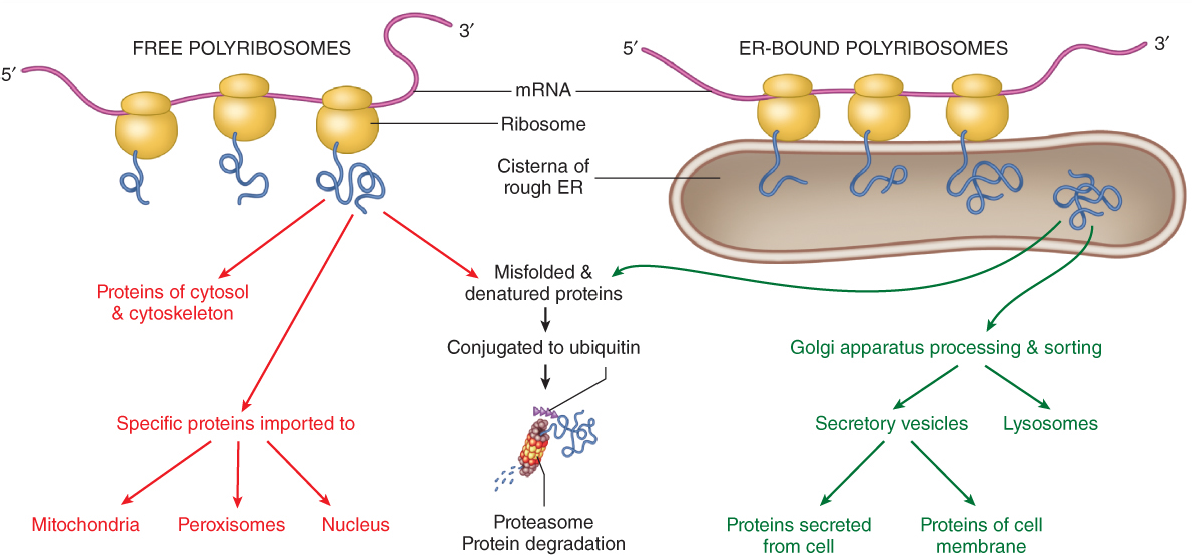
SLO 4. Explain how proteins and lipids are packaged into carrier vesicles, and how carrier vesicles fuse with target membranes including the plasma membrane.
Transport vesicles are membrane-delimited containers that carry lipids, integral membrane proteins, and solutes (including proteins) between organelles, and between organelles and the plasma membrane. We can outline in general how transport vesicles operate. Fig. 6 outlines the general themes in vesicular transport.
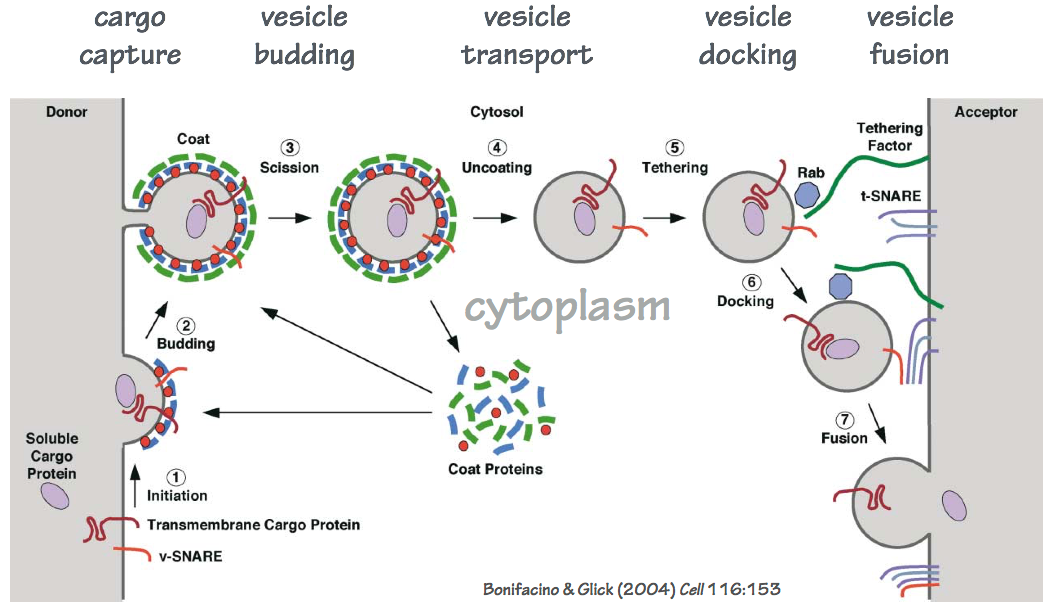
- Cargo lipids and proteins are identified by cytoplasmic coat complexes. Note that soluble secretory proteins must be identified by transmembrane receptors that traverse the membrane to engage with the coat complex. Note: different coat complexes assemble transport vesicles with different contents.
- The coat complex forms a shell that both captures cargo (lipids, proteins), and deforms the membrane (budding).
- The coated transport vesicle detaches from the donor membrane.
- The coat dissociates.
- Proteins and lipids on the transport vesicle are recognized by tethering factors that attach the transport vesicle to an acceptor membrane. We can think of these as molecular “Velcro” – but this “Velcro” has specificity: only appropriate vesicles will tether to a given acceptor membrane. Note: Different vesicles and acceptor membranes use different tethering factors.
- Tethering leads to a tighter association: docking. During docking, the fusion complex is assembled. The fusion complex contains SNARE proteins.
- Zippering of SNARE proteins is energetically favorable, and leads to fusion of the vesicle and acceptor membranes. Different combinations of SNARE proteins can assemble. Only some of these actually trigger fusion — an additional layer of specificity.
Here is an example of two specific pathways, operating in concert. If you examine this diagram closely, you’ll see specific examples of all the general mechanisms described on the previous page.
- The forward (anterograde) pathway uses the COPII coat to make vesicles that carry secretory cargo molecules from the ER to the cis-Golgi.
- The backward (retrograde) pathway uses the COPI coat to retrieve SNARE proteins and cargo receptors such as K/HDEL back to the ER. The retrograde pathway also retrieves mis-folded proteins that have failed quality control checks in the Golgi.
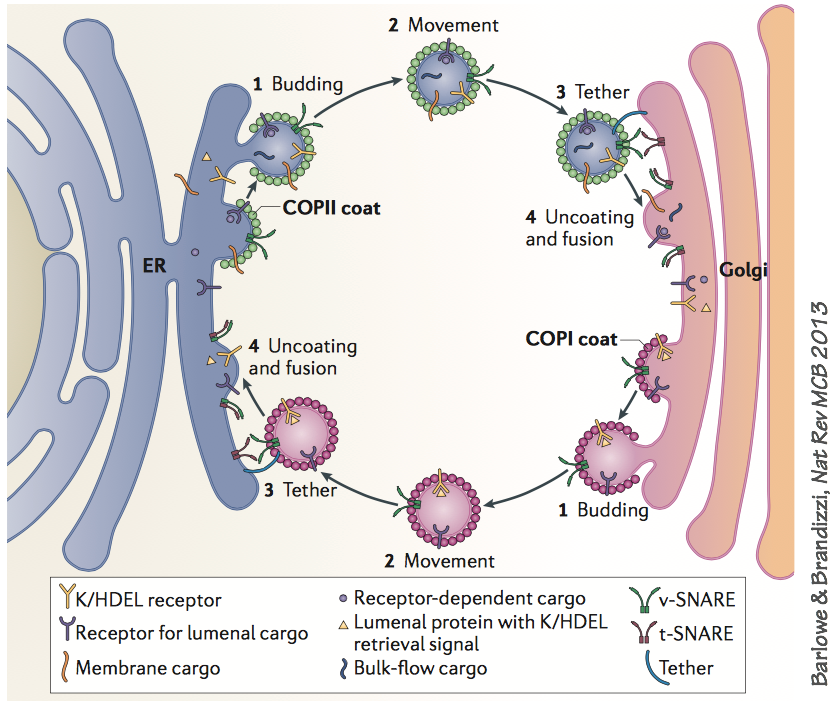
SLO 5. Explain key mechanisms that underlie endocytosis, receptor recycling, and traffic to the lysosome.
If you imagine how the secretory pathway operates in isolation, there is a problem: membrane is continuously deposited on the plasma membrane, but not retrieved. The plasma membrane grows and grows. Thus, there is a second system that brings membrane back into the cell: the endocytic pathway.
The endocytic system also has a degra
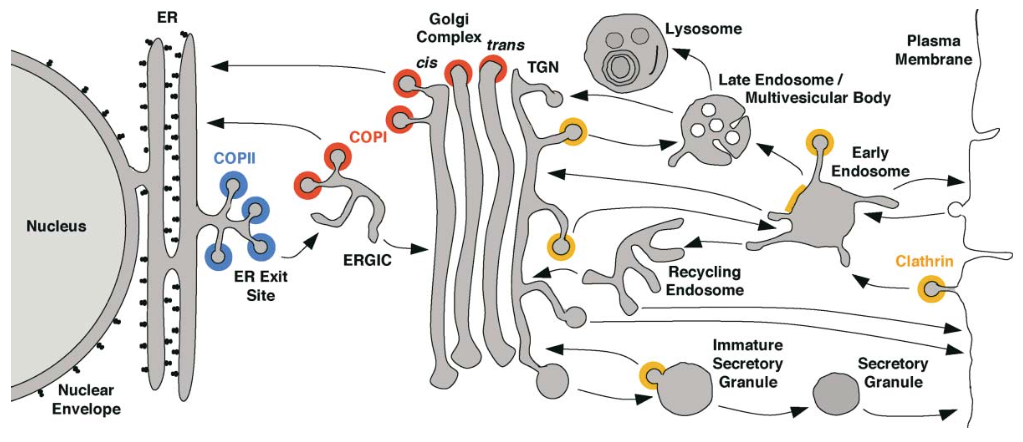
dative role, bringing membrane lipids, proteins, and surface- adsorbed particles into the cell, where they can be routed back into the secretory pathway or, alternatively, sent to the hydrolytic l
ysosome for destruction and recycling. The endocytic system also has a recycling pathway, as we’ll see below.
While the secretory pathway begins at the ER and ends with exocytosis (exit or ejection from the cell), the endocytic pathway begins at the plasma membrane with endocytosis (taking into the cell).
In endocytosis, the coat consists of an inner shell that recognizes cargo and an outer shell that organizes membrane deformation. The outer shell complex, clathrin, also operates at other steps of intracellular transport (Fig. 9). The inner shell adaptor system is diverse, with molecules specialized for capturing different cargo at different locations in the cell.
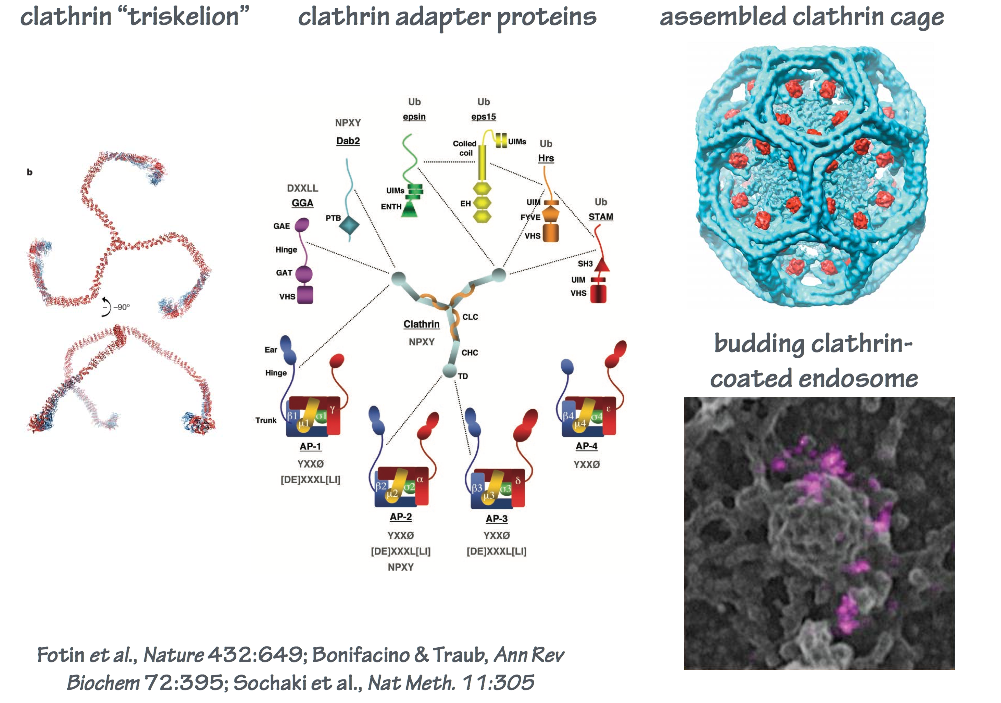
SLO 6. Outline the synaptic vesicle cycle, and explain how clostridial neurotoxins selectively block neurotransmission.
At chemical synapses, vesicles loaded with neurotransmitter fuse with the plasma membrane of the presynaptic cell. This is a regulated exocytosis event. This is followed by endocytosis.
Together, these events constitute the synaptic vesicle cycle. This is an example of an endocytic recycling pathway: the donor membrane from which the vesicle is derived, and the acceptor membrane with which the vesicle fuses, is the same membrane — the plasma membrane.
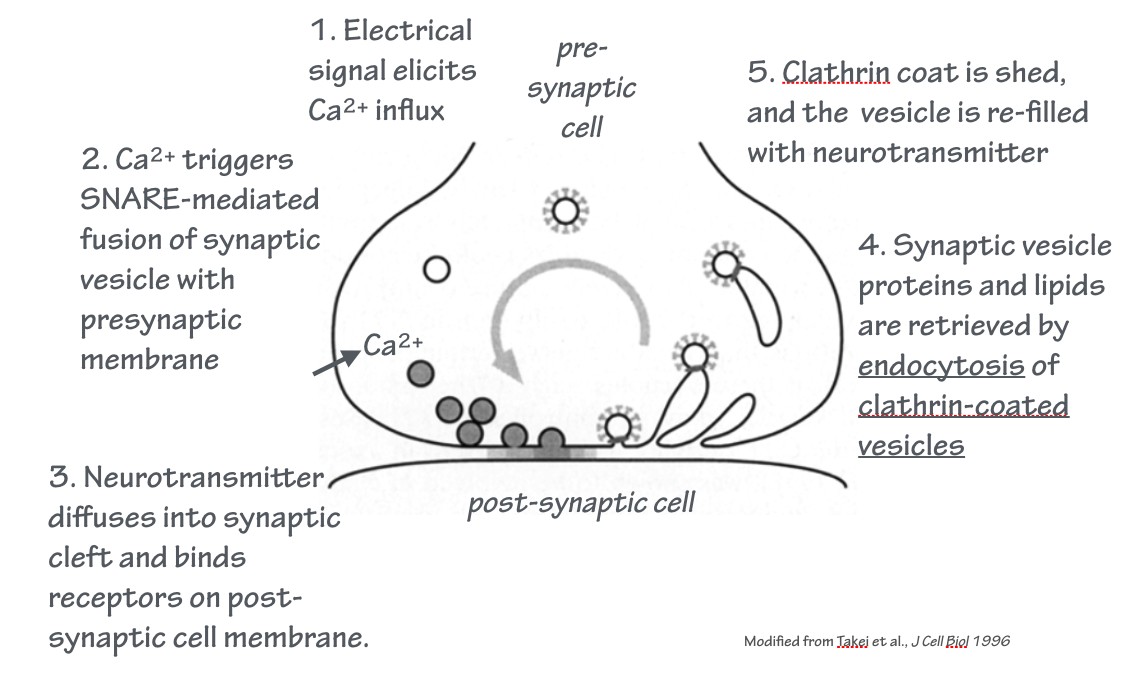
Clostridial neurotoxins are secreted by bacteria of the genus Clostridium, including the species that cause botulism and tetanus.
The clostridial neurotoxins bind to receptors on the plasma membrane, are endocytosed, and then the active toxin “payload” is translocated across the membrane into the cytoplasm. The active toxin is a protease enzyme that requires a bound Zn2+ metal ion for activity (a zinc protease).
These proteases have exquisite selectivity: they recognize only SNARE proteins involved in exocytosis, and cleave them. This potently blocks membrane fusion and neurotransmitter release — and, therefore, blocks neurotransmission (see Fig. 6).