12 Moving Energy
What determines the equator to pole temperature gradient on Earth, and how could it change? Circulations in the atmosphere and ocean bring a tremendous amount of heat polewards, amounting to almost 6 PW (6×1015 W) at the latitude of maximum transport, around 35 degrees north and south latitude. This transport evens out quite a bit of the sunlight gradient that heats the tropics so much more than the higher latitudes.
Many expect the ocean circulation to transport more heat than the atmosphere, but it’s quite the opposite. Around 4 PW is transported poleward by the atmosphere in each hemisphere. The oceanic transport reaches about 2 PW in the Northern Hemisphere (NH), and only a little over 1 PW in the Southern Hemisphere (SH). The oceanic divergence is larger in the deep tropics, and is associated with the large upwelling near the equator, and fluxes of energy associated with the subtropical cells.
Figure by Aaron Donohoe
The atmospheric energy transport can be decomposed into several components. The flux of dry static energy, [latex]s = c_p T + gz[/latex] represents the internal and gravitational potential energy that is transported, with cp the specific heat of dry air at constant pressure, T the temperature, g=9.8 m/s2 the gravitational acceleration, and z the height. Latent energy also contributes to the poleward transport of heat, and comes into the moist static energy [latex]m=c_p T + gz + L_v q[/latex], with Lv = 2.5 x 106 J/kg the latent heat of vaporization and q the specific humidity. Latent energy is an important component of the poleward transport of energy. When water vapor is evaporated in the subtropics, it requires energy. If the moisture is transported polewards in a baroclinic eddy, before condensing out at higher latitudes, it releases its latent energy where the condensation occurs. Thus energy is transported poleward by the movement of water vapor, just like when warmer air moves poleward and is mixed. Additional latent energy is released when freezing occurs, but this is smaller than the condensational heating. The transport of kinetic energy is much smaller compared with the other components.
The dry static energy transport and latent energy transport are each more complex in their latitudinal structure than their sum. Let’s consider the northward moisture transport first. It is southward in the SH extratropics, northward in the SH tropics, southward in the NH tropics, and northward in the NH extratropics. In other words, the transport is poleward in the extratropics and equatorward in the tropics (with the exception of a small northward flux across the equator). The equatorward transport of latent energy within the tropics is due to the Hadley circulation, which transports water vapor in the lower troposphere by its converging winds. In the extratropics, eddies carry filaments of high-moisture air poleward within weather systems. Such high moisture filaments are visible at any given instant in satellite data such as MIMIC-TPW.
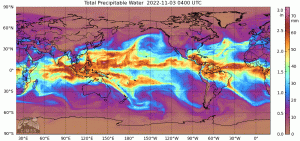
Consider the northward transport of dry static energy as determined by these same two processes: the Hadley circulation within the tropics, and eddies within the extratropics. In the Hadley circulation, poleward transport of dry static energy is very large, enough to offset the moisture transport completely and make the total atmospheric transport poleward (with the exception of the small southward flux near the equator). This is due to the high dry static energy air in the upper troposphere, where the air moves poleward. The poleward transport by the Hadley circulation means the circulation is thermally direct, i.e., it transports energy from warm to cold. Within extratropical weather systems, warm air is carried poleward and cold air equatorward, resulting in a poleward transport. Hence the latent and dry energy transports are of the same sign in the extratropics.
How Increasing Water Vapor Affects Transports
How could energy transports change with global warming? Since moisture content increases so rapidly, the latent energy transport should amplify in place, to first approximation. As described in the previous chapter, this is another “thermodynamic change” that occurs without any change in circulation. Imagine the same strength of converging winds within the lower branch of the Hadley circulation; these would carry more moisture equatorward in a more humid world. Similarly, a given strength of winds within extratropical storms would carry more moisture poleward.
In the Hadley cell, the dry static energy transports should increase substantially in response to the increased equatorward latent energy transport. As the atmosphere warms, temperatures increase the most in the upper tropical troposphere and the tropopause rises as well, as we showed in Chapter 9. Thus even with the same Hadley circulation winds, the dry static energy carried poleward will increase. Since latent heating determines the energy increase aloft, the sum of these two processes results in a small total atmospheric flux change. Next chapter we’ll discuss how a cross-equatorial Hadley circulation can appear when one hemisphere is heated more than the other.
Within the extratropics, the increase in moisture transport with global warming causes more latent heat release at high latitudes. This warms higher latitudes more, and decreasing the temperature gradients. So part of the tendency of the higher latitudes to warm more than the tropics is due to the increased moisture transport!
The total atmospheric transport responds to the altered gradients. Since temperature gradients have weakened, each midlatitude storm system brings a little less energy poleward. Hence the total atmospheric energy transport tends to change little in midlatitudes as well.
In climate models, energy transports tend to respond to patterns of heating, spreading an anomaly from one region around the planet. This diffusion of heating happens rapidly around latitude circles since east-west winds on Earth are especially strong. Diffusion of energy in the north-south direction is less rapid but still strong. What happens in one region of the planet is felt all around the world, via changes in transport of energy. Indeed, we live in a connected world.