4 Summation of Synaptic Potentials
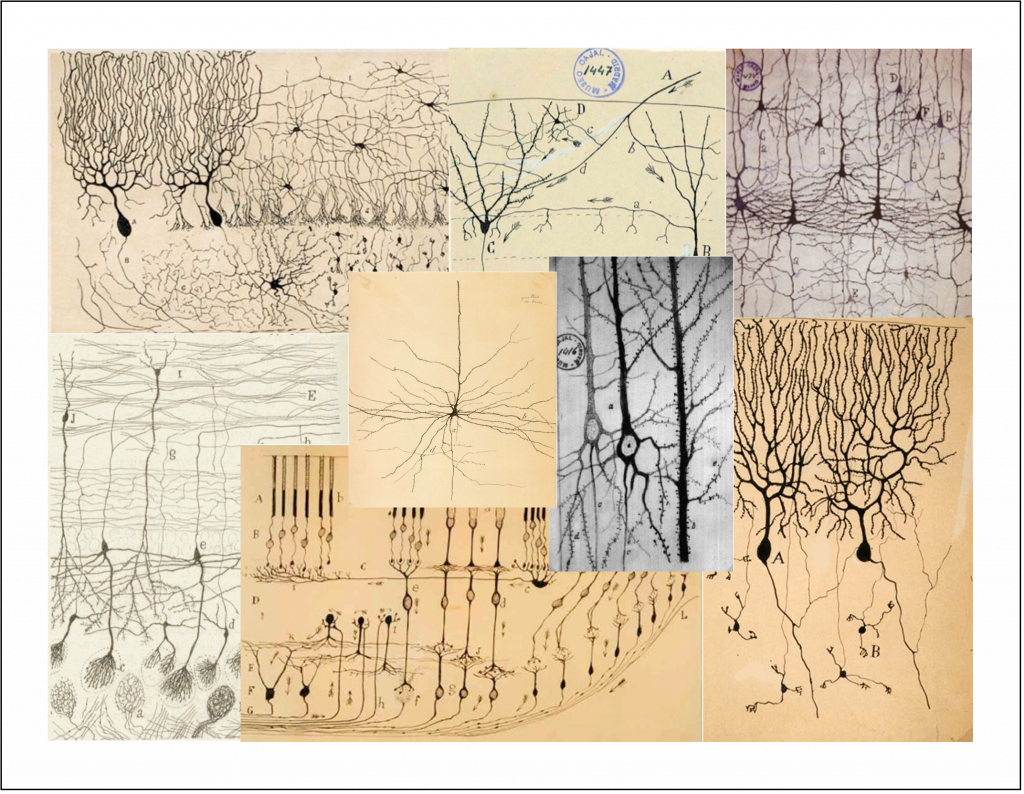
An important difference between synapses in the central nervous system and those at the neuromuscular junction is the number of excitatory inputs required to generate an action potential in the postsynaptic cell. At a healthy neuromuscular junction, a single presynaptic action potential reliably leads to an action potential in the muscle. A typical central nervous system neuron receives input from several thousand different neurons (convergence) and sends its outputs to several thousand neurons (divergence). These inputs are spread across the dendrites of the cells. You can appreciate the remarkable range of dendritic structures in Figure 6; these different structures are specialized for the precise function of the cell. Because the amplitude of a single synaptic input is typically less than 1 mV, multiple excitatory inputs must be summed or integrated to depolarize a neuron in the central nervous system to the firing threshold for generating an action potential. The term synaptic integration refers to the process by which multiple inputs are combined to control activity in the postsynaptic cell. This integration takes two forms:
• Temporal integration: integration of synaptic potentials from repetitive activation of one synaptic input. If the time between action potentials in the presynaptic cell is shorter than the duration of the synaptic potential produced in response to a single presynaptic action potential, the synaptic potentials produced by each action potential will begin to pile up, resulting in a larger response than that produced by a single presynaptic action potential. This process is referred to as temporal integration inputs originating at different times are combined or integrated.
• Spatial integration: integration of synaptic potentials from different synaptic inputs. Synaptic potentials produced at similar times from different synapses will be combined in the dendrites or at the soma of the postsynaptic cell and thus produce a larger response than that produced by activation of a single synapse. This is referred to as spatial integration because the inputs occur at similar times but at different spatial locations.
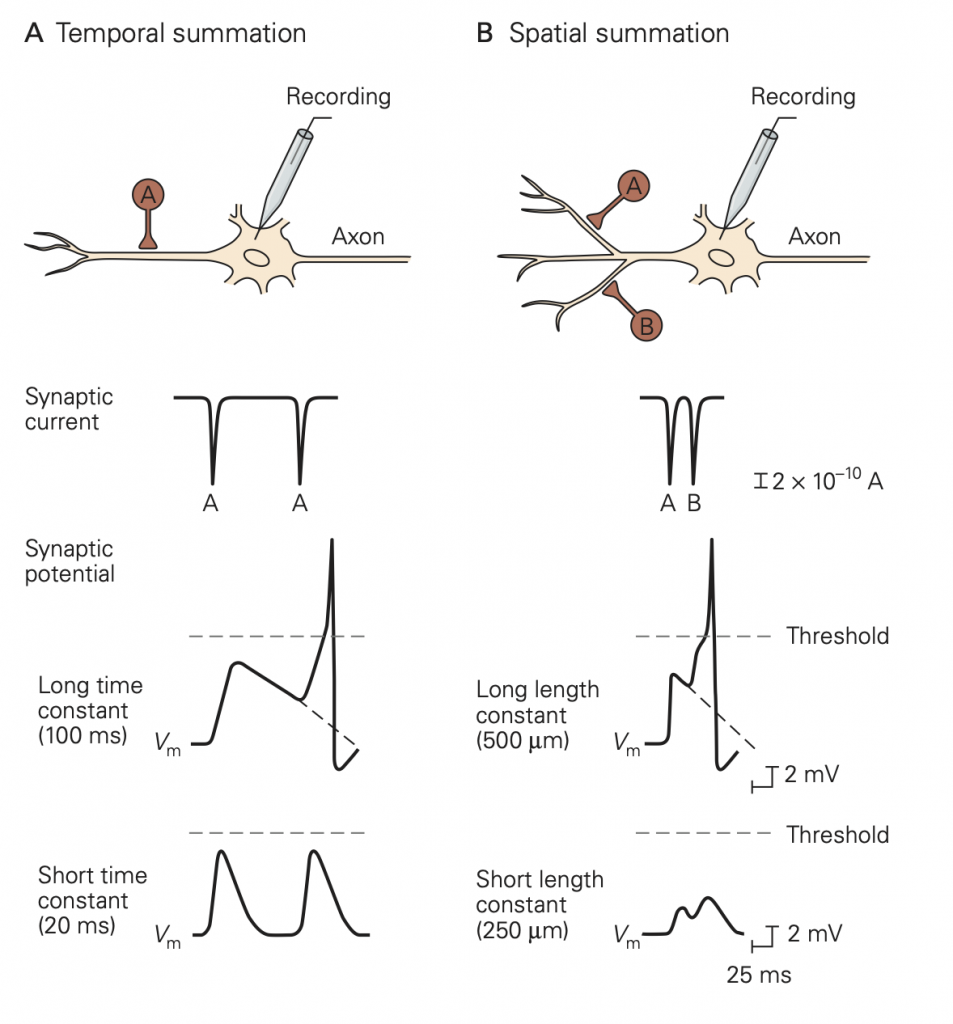
ASIDE: Active properties of dendrites
The discussion of temporal and spatial integration above focuses on dendrites as passive structures — i.e. the dendrites collect synaptic inputs and the resulting voltage changes spread passively to the soma where they are integrated. Although this is the classic view of dendrites, voltage-dependent ion channels in the dendrites can amplify or attenuate synaptic signals. Dendrites of some cells even express a sufficient number of voltage-activated Na+ channels to generate action potentials. It is unclear presently how generally important active properties of dendrites are for synaptic integration, though there are now a few examples in which they have a clear impact on neural function. This is a very active research area.
Pre- and post-synaptic inhibition
The massive convergence of inputs to a typical central nervous system neuron raises a conundrum: how does a cell receiving thousands of inputs (even if those inputs produce synaptic potentials less than 1 mV) maintain a reasonable firing rate? Fortunately, not all the inputs are excitatory, and indeed balancing excitatory and inhibitory inputs is critical to normal neural function. You can consider the neuron as a polling station where many voters are casting Yea and Nay votes to control action potential firing. The inhibitory signals that prevent runaway excitation are provided by inhibitory interneurons. These cells influence synaptic integration in two main ways.
• Presynaptic inhibition (Figure 8). In this case, activity in an inhibitory neuron (green in Figure 8) leads to activation of inhibitory synapses (which release GABA in the figure) made onto the presynaptic terminal (yellow). This activity reduces the amount of transmitter released by an action potential that subsequently propagates down the axon of the yellow cell and invades its synaptic terminal. A common mode of action is that the released inhibitory transmitter activates GPCRs, which initiate a G-protein cascade that inhibits presynaptic Ca2+ channels, causing fewer Ca2+ channels to open when the terminal is depolarized. This mode of inhibition requires that the (green) inhibitory neuron be active (i.e. generates an action potential) first, so that the green inhibitory synapse can be activated and exert its effect before the action potential arrives in the yellow synaptic terminal.
• Postsynaptic inhibition: Postsynaptic inhibition is a form of spatial integration: IPSPs generated by inhibitory inputs to a cell will fully or partially cancel EPSPs generated by excitatory inputs when they are combined in the dendrites or at the soma. Balancing of excitatory and inhibitory inputs in this context means that the average of all the EPSPs received by the cell tends to be cancelled by the average of all the IPSPs. Appropriate stimuli can disrupt this cancellation and cause either the EPSPs to win out, and the firing rate to increase, or the IPSPs to win out, and the firing rate to decrease. Thus, firing patterns of many neurons in the brain reflect changes in the balance of an ongoing barrage of excitatory and inhibitory synaptic inputs. As in a voting booth, the YES (excitatory) votes have to exceed the NO (inhibitory) votes for activity to increase. These balanced choices underlie the decisions made by your brain.
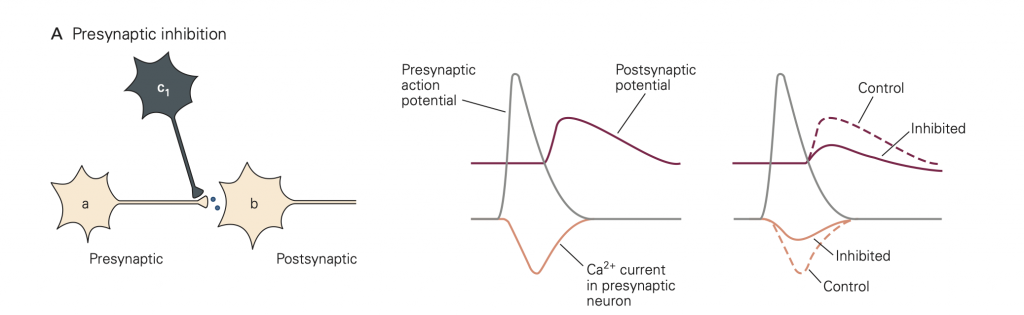
Aside
Disruption of the balance between excitatory and inhibitory inputs is one likely cause of diseases marked by strong bouts of abnormal neural activity, such as epilepsy. One possible treatment for such conditions is an increase in the strength of inhibitory inputs — for example by drugs such as benzodiazepines. Other approaches to achieve an increase in inhibitory input and restore a balance of excitation and inhibition are also being pursued — even transplanting neuronal precursor cells that differentiate into inhibitory interneurons!