1 Neurotransmitter Release
Basic sequence of events: The mechanisms governing transmitter release were first revealed in studies of neuromuscular junction. The neuromuscular junction is the chemical synapse formed by a motoneuron innervating a skeletal muscle fiber (Figure 1). While this synapse has several unique features, it also exhibits many general features of chemical synapses, and indeed was the place where many of these general features were first discovered. The basic sequence of events at neuromuscular junctions is as follows (Figure 2):
1. A nerve action potential – a large, rapid and discrete change in voltage – propagates down the axon of the motoneuron and depolarizes its synaptic terminal.
2. The presynaptic depolarization due to the action potential causes voltage-gated Ca2+ channels to open and to permit Ca2+ to enter the synaptic terminal.
3. Ca2+ entry is the signal to trigger exocytosis of presynaptic vesicles, which in motoneurons are filled with acetylcholine (ACh).
4. Released ACh quickly diffuses across the narrow synaptic cleft to the skeletal muscle fiber where it binds to nicotinic ACh receptors on the muscle membrane. These receptors are non-selective cation channels having about equal permeability to K+ and Na+. Binding of ACh opens these ACh receptor channels, leading to a local depolarization of the muscle called the end-plate potential. Under normal circumstances, the large synaptic depolarization always reaches firing threshold and gives rise to a muscle membrane action potential that propagates in both directions in the muscle.
Each action potential causes the muscle fiber to contract. Diseases that target the neuromuscular junction, for example myasthenia gravis, can reduce the end-plate potential so it sometimes fails to elicit an action potential in the muscle. Such failures manifest as muscle weakness.
5. ACh is removed from the synaptic cleft both by enzymatic degradation (by acetylcholinesterase) and by diffusion.
Neurotransmitter release: Classic studies at the neuromuscular junction by Bernard Katz and colleagues revealed two features of neurotransmitter release that are shared by all chemical synapses (Figure 2):
(1) The chemical neurotransmitter is stored in presynaptic vesicles.
(2) Intracellular Ca2+ is the trigger signal for neurotransmitter release.
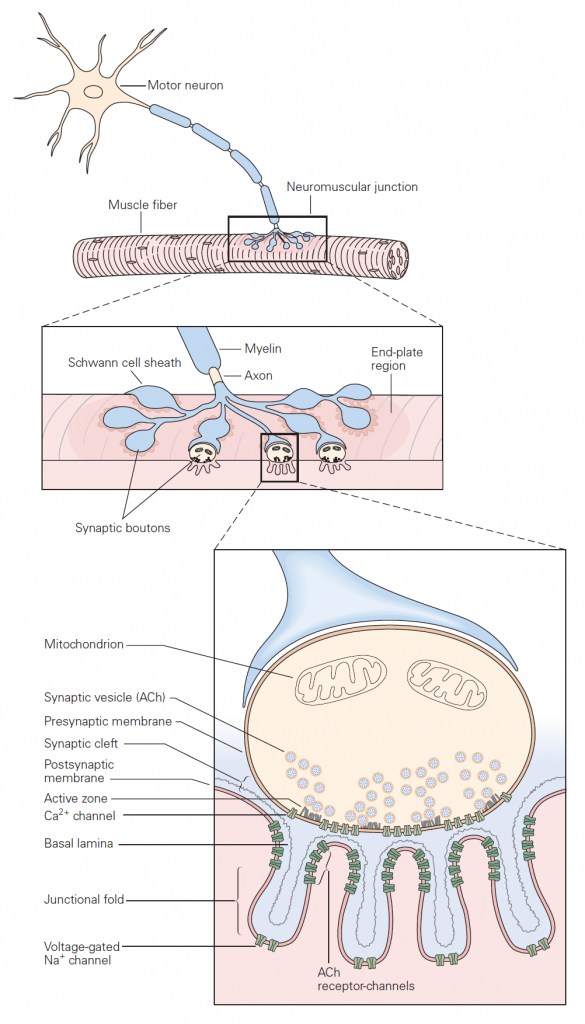
When an action potential propagates into the synaptic terminal, the resulting depolarization leads to the opening of voltage-gated Ca2+ channels and Ca2+ entry into the synaptic terminal. Like voltage-gated Na+ and K+ channels that produce action potentials, these voltage-gated Ca2+ channels open in direct response to depolarization. They contrast with ligand-gated channels that open due to the binding of a chemical ligand (e.g. the ACh channels expressed on the muscle at neuromuscular junction). The influx of Ca2+ and the resulting increase in internal Ca2+ concentration acts on proteins that control exocytosis. You have heard about these SNARE proteins already (see the class on Cell biology of secretion); they are a key part of conserved machinery involved in the release of chemical neurotransmitters. Ca2+-induced conformational changes in these proteins lead to the fusion of synaptic vesicles with the presynaptic cell membrane; neurotransmitter stored in the vesicles can then diffuse across the synaptic cleft to act on receptors in the postsynaptic cell (see below). The increase in internal Ca2+ is a requisite signal to initiate neurotransmitter release.
These properties — storage of transmitter in vesicles and the requirement for Ca2+ in triggering vesicle fusion — are conserved across all chemical synapses. Further, the protein machinery involved in exocytosis is largely conserved across synapses and is the target of several toxins such as botulinum and tetanus toxins. Botulinum toxin causes flaccid muscle paralysis by cleaving SNARE proteins and hence rendering presynaptic motoneurons incapable of transmitter release. In cosmetic medicine, this toxin under the name Botox is used to relax wrinkles caused by contraction of facial muscles.
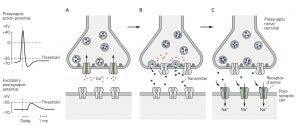
the postsynaptic cell compared with the virtually instantaneous transmission of signals at electrical synapses (see Figure 8–2B).
A. An action potential arriving at the terminal of a presynaptic axon causes voltage-gated Ca2+ channels at the active zone to open. The gray filaments represent the docking and release sites of the active zone. B. The Ca2+ channel opening produces a high concentration of intracellular Ca2+ near the active zone, causing vesicles contain- ing neurotransmitter to fuse with the presynaptic cell mem- brane and release their contents into the synaptic cleft
(a process termed exocytosis). C. The released neurotransmitter molecules then diffuse across the synaptic cleft and bind specific receptors on the postsyn- aptic membrane. These receptors cause ion channels to open (or close), thereby changing the membrane conductance and membrane potential of the postsynaptic cell.
Diversity of central synapses
The neuromuscular junction is a highly specialized giant synapse — a one-to-one contact in which each action potential in the nerve is faithfully transmitted to the muscle under normal circumstances. Synapses between neurons in the nervous system show considerably more diversity. This diversity arises from differences in the identity of the released neurotransmitters, differences in the type of receptors that the neurotransmitters act on, and differences in how multiple synaptic inputs are combined or integrated by the postsynaptic cell. Below we consider each of these issues in more detail.
Postsynaptic receptors at central synapses
At all chemical synapses, as at the neuromuscular junction, released chemical transmitter diffuses across the synaptic cleft and binds to and activates receptors on the post-synaptic cell. Receptor activation leads to a conductance change (almost always a conductance increase, due to opening of ion channels), and this in turn leads to a synaptic potential in the postsynaptic cell. The synaptic potential is a local, graded change in membrane voltage analogous to the end-plate potential at neuromuscular junctions. In the CNS, the synaptic potential from a single excitatory nerve synapse is typically quite small and would not by itself initiate an action potential in the postsynaptic cell (see discussion of integration below). In this respect most synaptic potentials are quite different from single end-plate potentials, which in normal muscle always lead to an action potential. As described below, synaptic potentials in the CNS can be depolarizing (excitatory postsynaptic potentials or EPSPs) or hyperpolarizing (inhibitory postsynaptic potentials or IPSPs). Key types of receptors mediating these different synaptic potentials are summarized in the following table.
Synaptic potential | transmitter | Receptor type | Predominant location |
Fast EPSP | glutamate ACh | NMDA, kainate, AMPA Nicotinic ACh receptor | Central nervous system Skeletal muscle autonomic ganglia |
Fast IPSP | GABA
glycine |
GABAA receptor Glycine receptor | Central nervous system Spinal cord |
Slow EPSP | ACh | Muscarinic ACh | Smooth muscle |
Slow IPSP | GABA
ACh |
GABAB receptor Muscarinic ACh receptor | Central nervous system
heart |
Table 1: Neurotransmitters mediating several key types of synaptic potentials. Fast synaptic potentials are mediated by ionotropic receptors and slow synaptic potentials by GPCR metabotropic receptors (see Learning Objective 3).