Nociception and Spinal Reflexes
John Tuthill
Summary: Nociception and Spinal Reflexes
Nociceptors are sensory neurons that respond to physical stimuli strong enough to cause tissue damage. They innervate every tissue of the body except, ironically, the brain. Nociceptors are classified by the type of physical stimuli (i.e., modality) that they respond to, such as pressure, heat, or cold. Their activity gives rise to the perception of pain.
A spinal reflex is a rapid activation of muscle produced by the stimulation of sensory receptors. Spinal reflexes are mediated by nerve cells in the peripheral nervous system (sensory neurons) and the spinal cord (spinal interneurons, cells confined to the spinal cord and motor neurons, the cells that innervate skeletal muscle). Unlike planned, intentional movements, reflexes are involuntary and can be evoked without the involvement of the brain.
The simplest spinal reflex is called the tendon jerk or knee jerk and it is widely used in neurological examinations to examine the integrity of sensory and motor functions of the spinal cord. This spinal reflex is mediated by actions of just two populations of neurons: the primary sensory receptors of a sense organ called the muscle spindle and motor neurons, the cells that activate skeletal muscle fibers.
Application of a noxious stimulus to the skin results in widespread and powerful activation of ipsilateral flexor muscles that generally produces a withdrawal of the limb away from the stimulus, called the flexion reflex. The opposite reaction, called the crossed-extension reflex, is often observed in the contralateral limb.
Lesions in the brain or spinal cord and several disease processes can be manifested in excessive spinal reflexes and spasticity.
Learning Objectives
- To know how nociceptors are activated and sensitized.
- To understand the difference between reflexes and other types of movements.
- To differentiate the neural circuits underlying the flexion and stretch reflexes.
- To understand how reflex testing can be used clinically to diagnose neuro-pathologies that affect motor and sensory function.
(Unless otherwise noted, all figures are from: Kandel ER, Schwartz JH, Jessell TM 2012, Siegelbaum SA, Hudspeth AJ. ‘Principles of Neural Science, 5th ed. McGraw-Hill, New York.)
Nociceptors are classified by the type of physical stimuli (i.e., modality) that they respond to. Some nociceptors respond to more than one modality and are referred to as polymodal nociceptors. Others, called sleeping or silent nociceptors, normally do not respond to any type of stimulus, but become activated under conditions of inflammation or tissue damage.
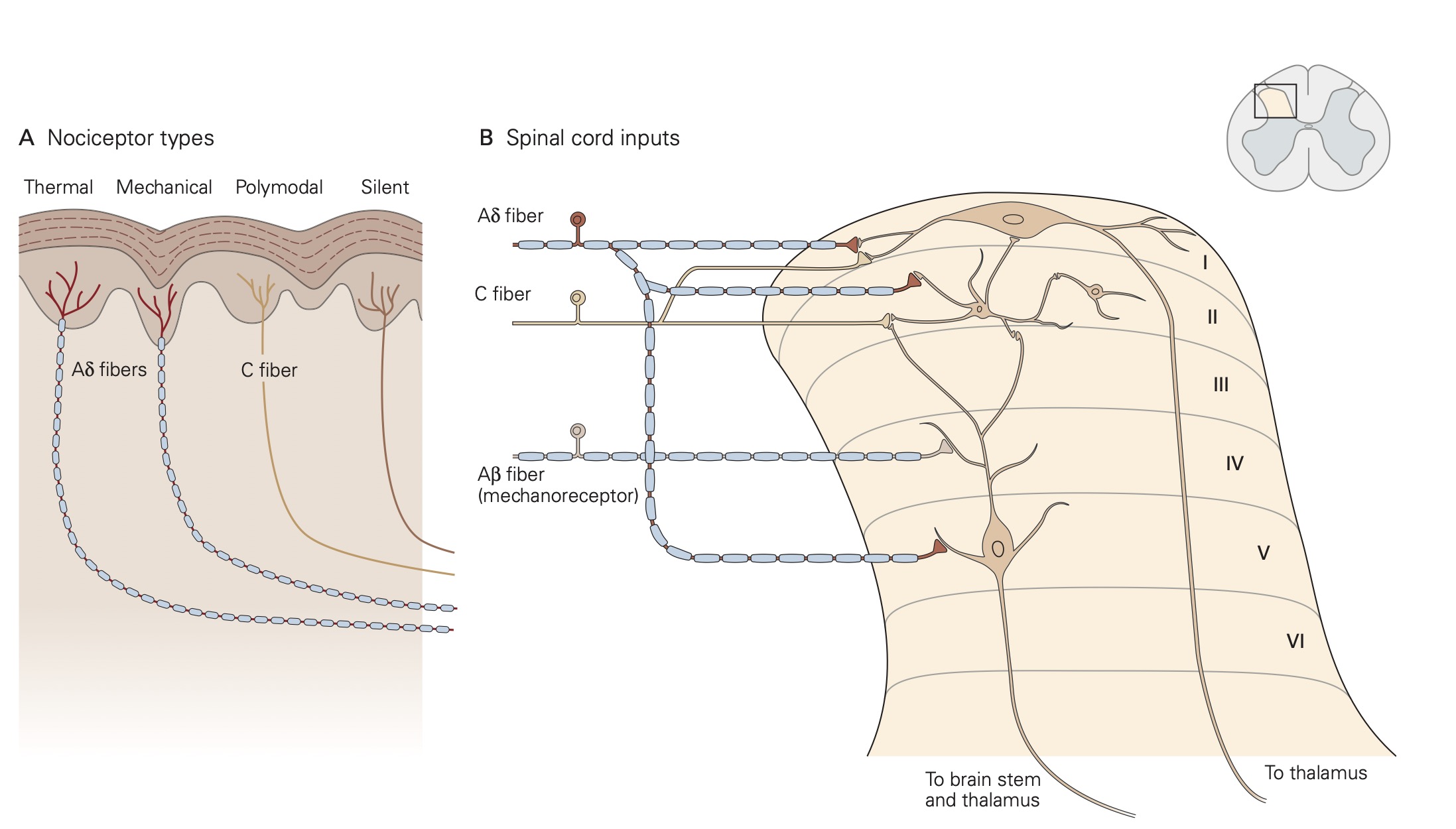
Thermal nociceptors are activated by noxious (i.e., tissue damaging) hot or cold temperatures within the receptive field acting on TRP channels. TRPV1 channels are expressed on thermal nociceptors that respond to heat/pain temperatures above 42 °C. Other temperatures in the warm–hot range are transduced by different thermal nociceptors that express a different TRP channel. Cold stimuli are sensed by thermal nociceptors that express TRPM8 channels. An interesting finding related to cold stimuli is that tactile sensibility and motor function deteriorate while pain perception persists.
Mechanical nociceptors respond to excessive mechanical deformation of the tissue they innervate. They also respond to wounds that break the epidermal surface. These mechanical nociceptors that also express TRP channels frequently have polymodal characteristics, responding to thermal stimuli and chemical stimuli.
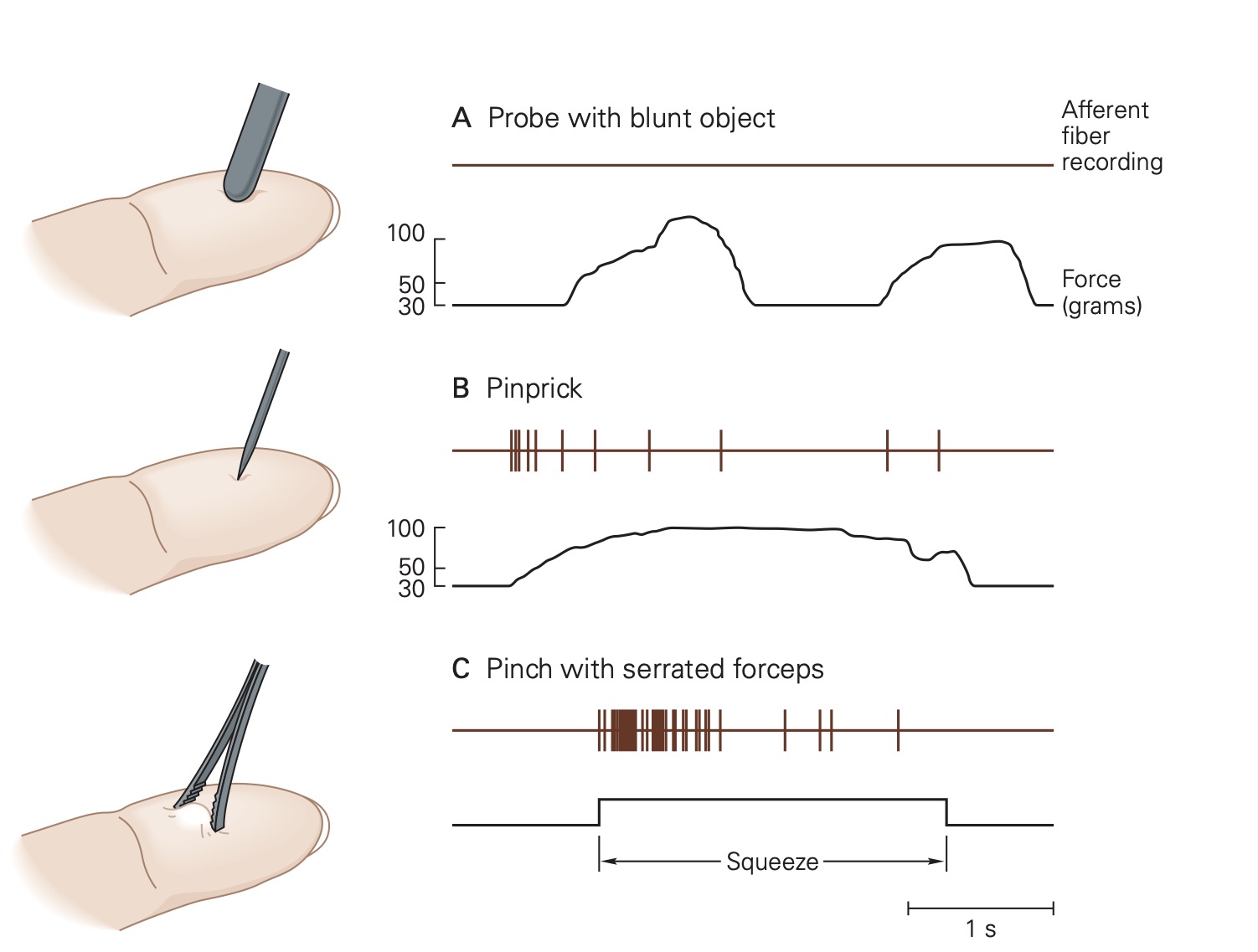
Chemical nociceptors express TRP channels that respond to a wide variety of molecules including spices like capsaicin (8-methyl-N-vanillyl-6-nonenamide), the active, hot sensation producing substance in chili peppers and environmental irritants like acrolein, a component of cigarette smoke. Chemical nociceptors also respond to endogenous ligands, and certain fatty acid amines that arise from damage to internal tissues.
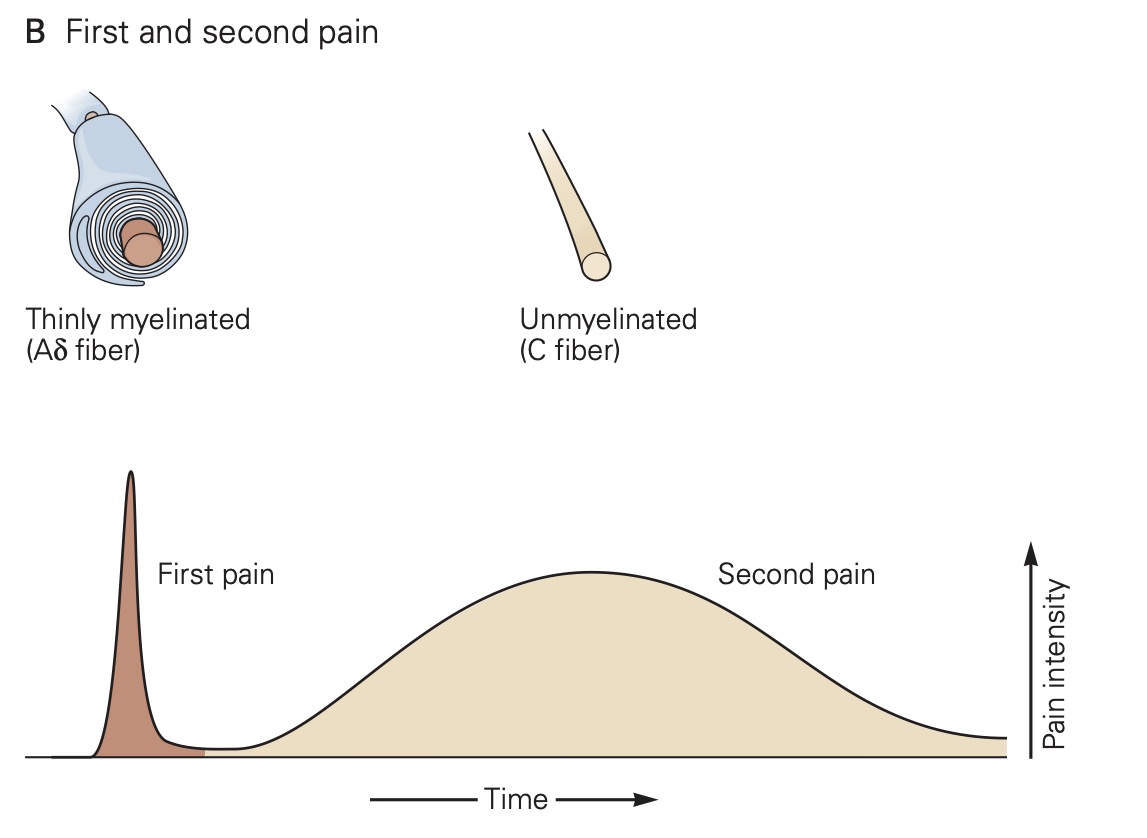
Individual nociceptors are grouped into two different classes, which have distinct types of axons: A-∂ fibers are myelinated and conduct action potentials quickly, within 10-30 m/s, while unmyelinated C-fibers conduct action potentials more slowly, from 0.5 – 2 m/s. Due to these differences in axonal conduction speed, nociception and the affective perception of pain often come in two phases: The first phase, initial sharp and localized pain, is mediated by the fast-conducting Aδ fibers and the second phase, continuous, long-lasting and more diffuse pain, is generated by activation of the slower- conducting C-fibers.
Response of nociceptors to tissue damage
Exposure to noxious stimuli often results in tissue damage. When this occurs, the plasma membrane of individual cells is breached and proteases are released. Proteases cleave proteins and produce peptides, including one termed bradykinin, a very potent pain-inducing substance. Cleaved bradykinin acts by binding to its receptors: B2 and B1. B2 receptors are constitutively expressed and are important in mediating pain detection and vasodilation. B1 receptors are upregulated in damaged tissue and play an important role in chronic pain. Bradykinin receptors on nerve terminals, especially free nerve endings, will depolarize in response to bradykinin binding.
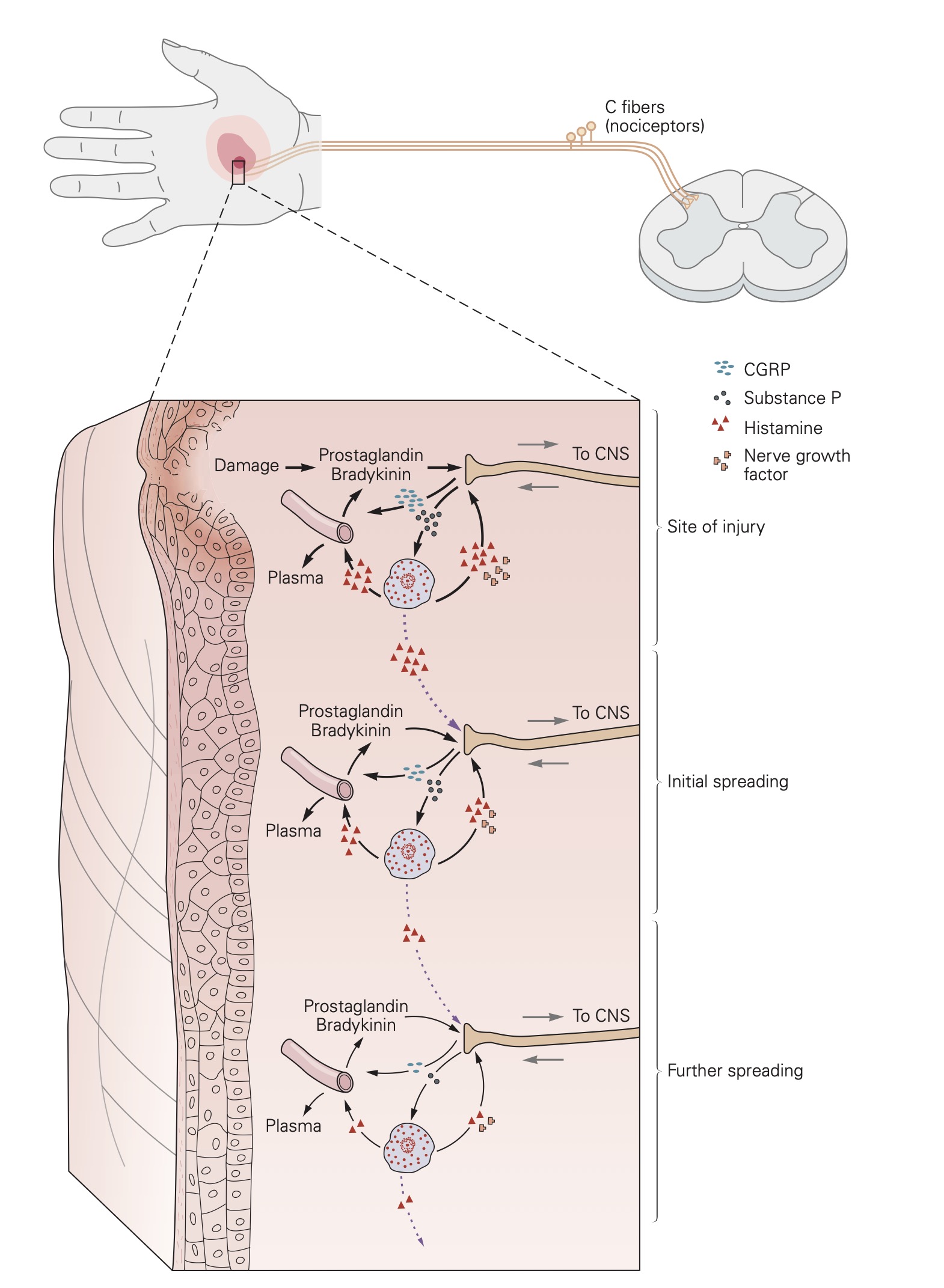
Sensitization of nociceptors
The responsiveness of nociceptors to stimulation can be modified, a process called sensitization, by a number of substances that interact with the receptive membrane. In the extreme, a nociceptor can be transformed from a noxious stimulus detector into one that responds to innocuous stimuli as well. When sensitized in this way, low intensity, innocuous stimuli associated with regular activity, can activate nociceptors and thus, generate a painful sensation. This is commonly known as hyperalgesia. Inflammation is one common cause of nociceptor sensitization. Normally hyperalgesia desists when the inflammation is resolved. Occasionally, however, repeated injury or chronic use of opioid analgesics can result in allodynia, a condition in which a completely non-noxious stimulus like light touch causes extreme pain. Allodynia can also be caused when nociceptor afferent fibers are damaged in the peripheral nerves.
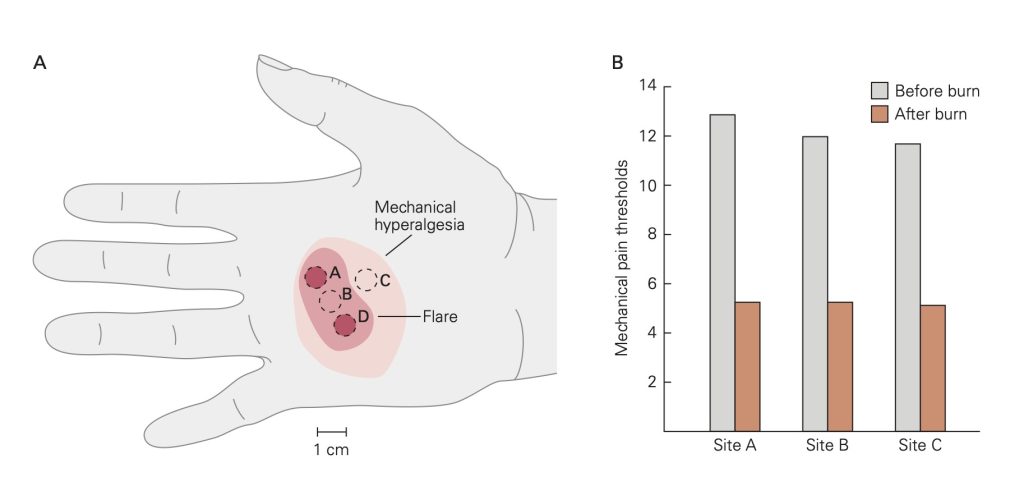
Learning Objective #2: To understand the difference between reflexes and other types of movements.
Learning Objective #3: To differentiate the neural circuits underlying the flexion and stretch reflexes.
Stimulation of nociceptors triggers a reflexive withdrawal of the affected body part away from the stimulus. A reflex is a rapid activation of muscle produced by the stimulation of sensory receptors. Spinal reflexes are mediated by nerve cells in the peripheral nervous system (sensory neurons) and the spinal cord (spinal interneurons, cells confined to the spinal cord and motor neurons, the cells that innervate skeletal muscle). Unlike planned, intentional movements, reflexes are involuntary and can be evoked without the involvement of the brain. Importantly, many reflexes are flexible and can be altered depending on body posture and behavioral context.
Flexion reflex
Application of a noxious stimulus to the cutaneous surface results in widespread and powerful activation of ipsilateral flexor muscles that generally produces a withdrawal of the limb away from the stimulus. One can also evoke a weak contraction of the same flexor muscles located in a given area by probing the skin with a sharp-pointed probe. Both of these responses are referred to as the flexion reflex.
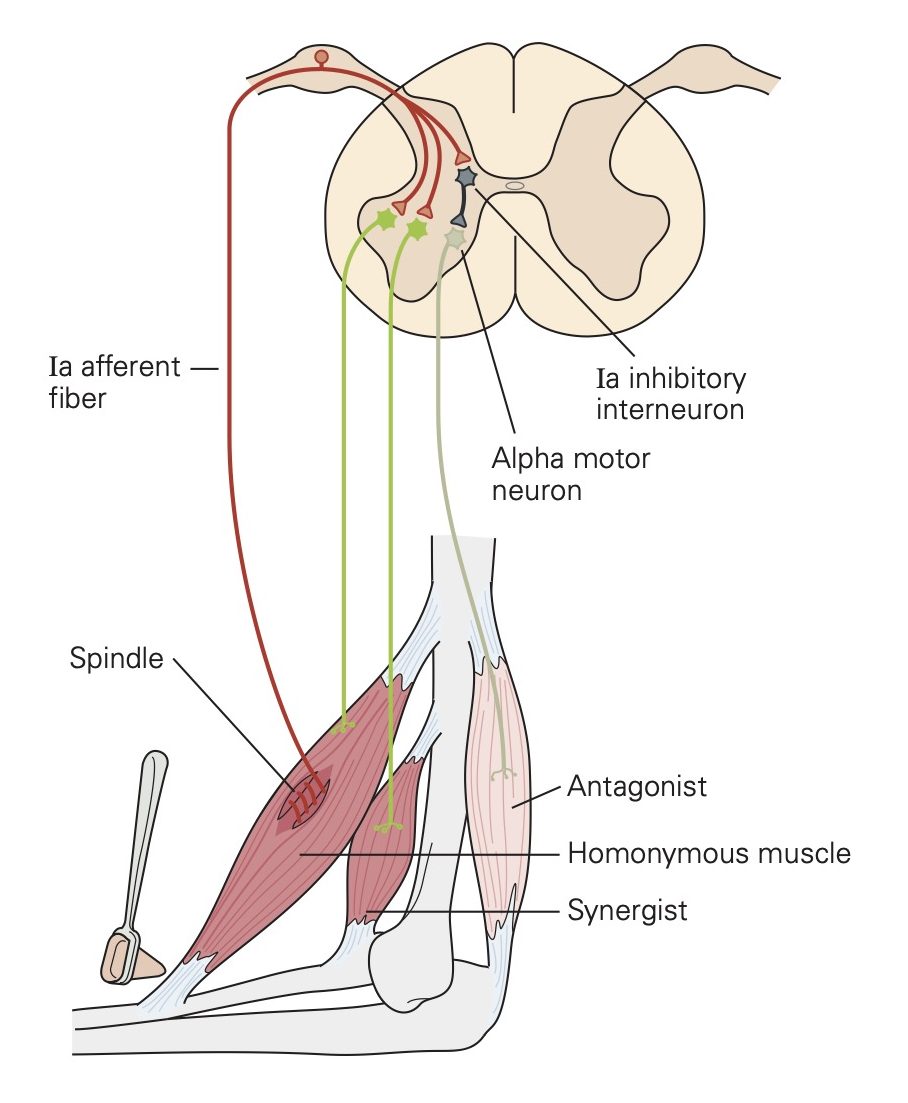
The reason that both noxious and innocuous stimuli can evoke flexion reflexes is that the sensory neurons associated with different modalities converge onto common interneurons in the spinal cord. The heterogeneous groups of sensory afferent fibers that evoke flexion reflexes are called the flexor reflex afferents (FRA). The FRA include A-b, A-∂ and C-fibers that innervate mechanoreceptors, chemoreceptors, temperature receptors and nociceptors found in virtually all of our tissues. The FRA generate excitatory postsynaptic potentials (EPSPs) that lead to action potentials in the spinal interneurons they contact synaptically. Some of these spinal interneurons generate EPSPs in flexor motor neurons and other spinal interneurons generate inhibitory post- synaptic potentials (IPSPs) in extensor motor neurons.
Crossed-extension reflex
Some of the interneurons that receive excitation (EPSPs) from the FRA send collaterals of their axons to the contralateral segment of the cord via the anterior commissure. These collaterals excite other interneurons which in turn influence the excitability of both flexor and extensor motor neurons. In general, the net effects are excitation (EPSPs) of contralateral extensor motor neurons and inhibition (IPSPs) of contralateral flexor motor neurons, often resulting in what is called the crossed-extension reflex. One might reasonably speculate that this reflex pattern evolved to facilitate the contralateral limb’s assuming additional weight bearing in the event of a limb withdrawal from a harmful stimulus.
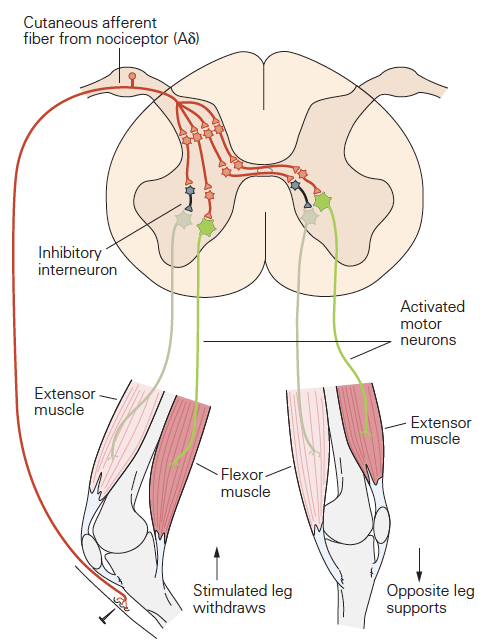
Learning Objective #4: To understand how reflex testing can be used clinically to diagnose neuro-pathologies that affect motor and sensory function.
Tendon-jerk/knee-jerk reflex
The simplest spinal reflex is called the tendon jerk or knee jerk and it is widely used in neurological examinations to examine the integrity of sensory and motor functions of the spinal cord. This spinal reflex is mediated by actions of just two populations of neurons: the primary sensory receptors of a sense organ called the muscle spindle and the a-motor neurons, the cells that activate skeletal muscle fibers. The neural circuit of the tendon jerk is outlined in the figure below.
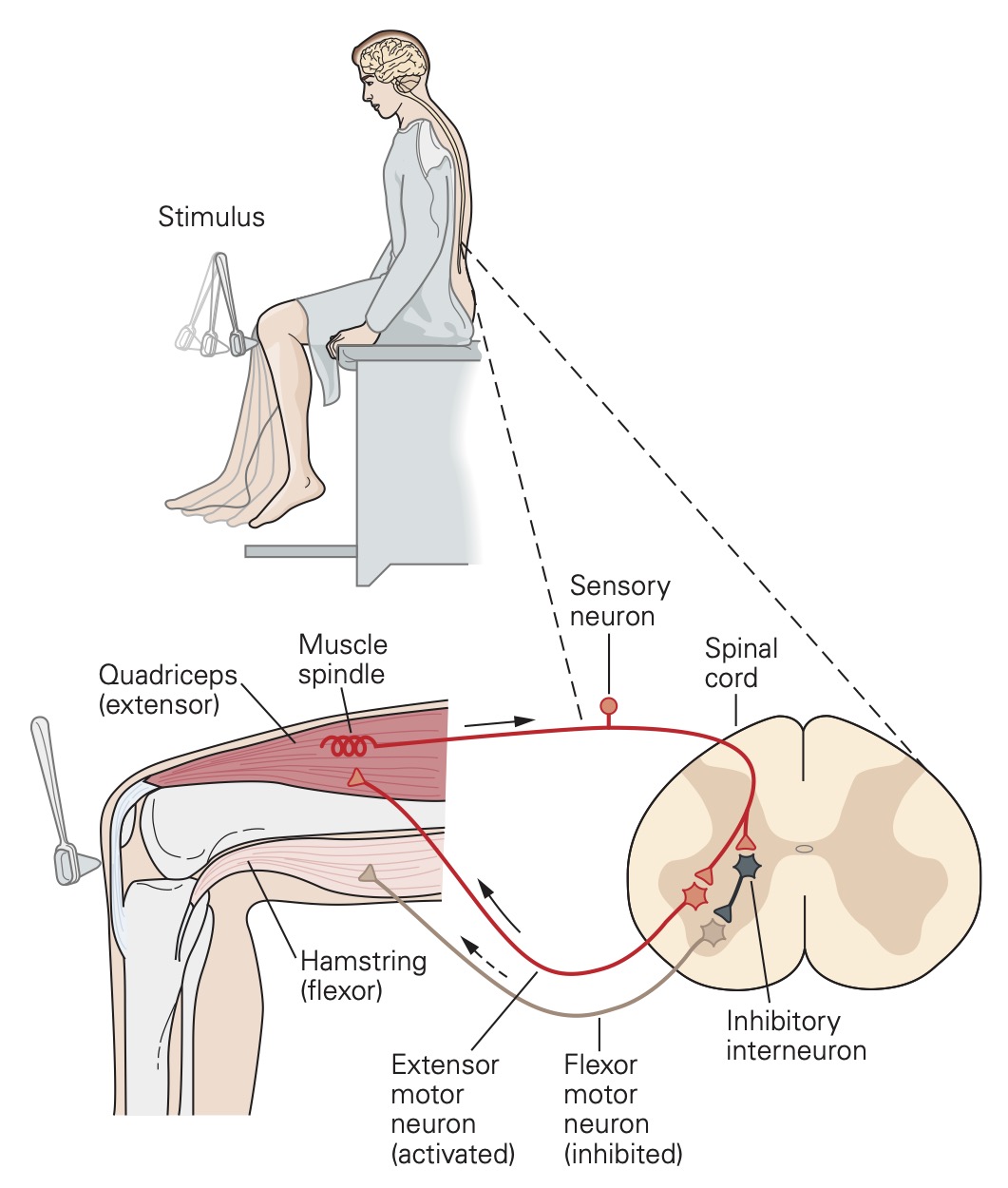
The tendon jerk reflex can be easily assessed in patients. Applying a brisk tap to the patellar tendon with a reflex hammer just below the knee normally results in a small contraction of the quadriceps muscles that extends or jerks the knee slightly. The mechanism underlying this reflex is as follows: The tendon tap produces a small, rapid stretch of the quadriceps muscles. Muscle stretch is the adequate stimulus for the primary or annulospiral sensory receptors of the muscle spindles. There are many muscle spindles scattered throughout the muscles, and each spindle has one annulospiral receptor. The muscle stretch evokes a depolarizing receptor potential from annulospiral receptors of the muscle spindles within the quadriceps muscles. These depolarizing receptor potentials result in a few action potentials that are propagated to the spinal cord by the group Ia sensory neurons that terminate as synaptic contacts, called ‘boutons’ on neurons in the spinal cord.
Each of the group Ia afferent fibers gives rise to many synaptic boutons in the spinal cord that contact all of the alpha motor neurons innervating the quadriceps muscles. The group Ia fiber synaptic boutons produce EPSPs in these alpha-motor neurons. The EPSPs depolarize some of these alpha motor neurons to threshold causing them to generate action potentials. The action potentials are then propagated to the muscle resulting in a twitch contraction that extends the knee.
The neural circuit mediating the tendon jerk is referred to as a monosynaptic pathway because there is only one synapse between the afferent (group Ia afferent fiber) and efferent (alpha motor neuron) elements of the circuit. Most neural circuits are multi- or polysynaptic, like the flexion and crossed- extensor reflex spinal circuits that were outlined earlier.
The same population of group Ia afferent fibers that produces the tendon jerk reflex also makes direct (monosynaptic) connections with alpha motor neurons that innervate synergist muscles, i.e., muscles that exert a similar mechanical action on the same joint. However, the group Ia afferent fibers make fewer contacts with these synergist motor neurons, such that the EPSPs generated in them are smaller than those generated in their own (called homonymous) motor neurons. The resulting depolarization in the synergist motor neurons fails to bring them to threshold and, thus, normally no reflex contraction occurs in the synergist muscles.
One can also measure tendon jerk reflexes by recording electromyographic (EMG) signals from muscles in response to a tendon tap. The EMG is a consequence of the action potentials generated by the active muscle fibers.
H-reflex
Group Ia afferent fibers can also be stimulated electrically in human subjects when the peripheral nerve in which they are contained lies just below the skin surface. In general, the group Ia afferent fibers are slightly larger in diameter than the axons of the a motor neurons in the same nerve and, thus, they have a lower threshold to an externally applied electrical shock. Applying a single shock through electrodes placed over the popliteal fossa, for example, produces a brisk reflex contraction of the soleus muscle, which closely resembles the response elicited by a tendon tap stimulus. This technique evokes what is called the H- or Hoffman Reflex. H-reflex testing is widely used both in the laboratory and the clinic. The H-reflex can be measured by recording an EMG signal from either surface electrodes or intramuscular electrodes. (As the strength of the electrical stimulus is increased, the H-reflex is preceded by an M-wave that results from direct activation of the a- motor neuron axons in the same peripheral nerve.)
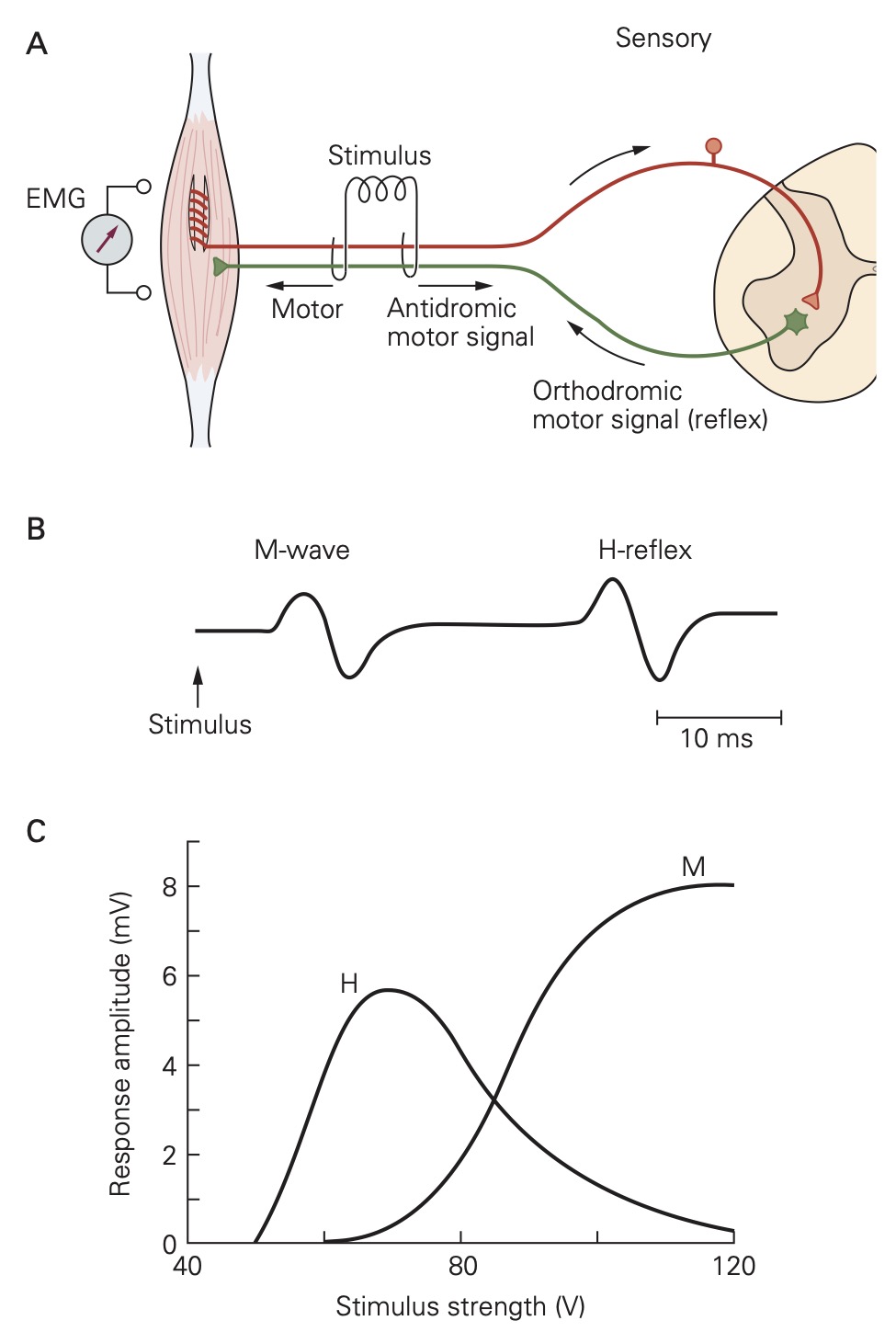
Reciprocal innervation
As a general rule, afferent fibers that excite one group of alpha motor neurons inhibit alpha motor neurons that innervate antagonist muscles. Antagonist muscles are those with opposing actions across a joint (i.e., flexion vs. extension; adduction vs. abduction). This common pattern has been referred to as reciprocal innervation.
The simplest inhibitory pathway is di-synaptic, that is, there are two synapses interposed between the afferent fibers and the a-motor neurons. For example, a Ia afferent fiber originating from a muscle spindle in a biceps muscle makes synaptic contacts with the biceps motor neurons and with a group of spinal interneurons in the spinal cord. (A spinal interneuron is a cell whose soma, axon and dendrites are contained completely within the spinal cord.) The synaptic boutons of the group Ia afferent fiber generate EPSPs in both the alpa motor neurons and these spinal interneurons. This excitation leads to the generation of action potentials in some of the interneurons. The interneurons in turn make synaptic contacts with alpha motor neurons innervating the antagonist triceps muscles. The interneurons produce IPSPs in the triceps motor neurons and, thus, inhibit the activation of these muscles. These interneurons are called Ia inhibitory interneurons because they are activated by group Ia afferent fibers and inhibit a-motor neurons.
Spasticity and the clasp-knife reflex
Spasticity is a condition characterized by hypertonicity (increased muscle tone), clonus (a series of rapid muscle contractions), exaggerated tendon jerk reflexes, involuntary muscle spasms, scissoring (involuntary crossing of the legs), and stiff joints caused by co-contraction of antagonist muscles. The degree of spasticity varies from mild muscle stiffness to severe, painful, and uncontrollable muscle spasms. Spasticity can interfere with rehabilitation in patients with certain disorders, and often interferes with daily activities. Spasticity is usually caused by damage to brain or spinal neurons involved in the control of movement. It may occur in association with spinal cord injury, multiple sclerosis, cerebral palsy, anoxia, brain trauma, severe head injury, and diseases such as adrenoleukodystrophy, amyotrophic lateral sclerosis (ALS), and phenylketonuria. Inferences drawn from an animal model suggest that one of the principal mechanisms of spasticity is an increase in the excitability of motor neurons consequent to the lesion in the brain or spinal cord. The increased excitability results in sustained repetitive discharge of a-motor neurons in response to brief stimuli.
The clasp-knife reflex (also called the inverse myotatic reflex) is a pathological reflex that is only observed following lesions of the central nervous system that produce spasticity. In response to a rapid muscle stretch, a patient with spasticity first exhibits a strong stretch reflex, followed by a relaxation of the muscle. It is the sudden release of muscle tension when a contracting muscle is forcibly stretched that gives the reflex its name: The spastic limb initially resists flexion (because of the stretch reflex) and then collapses on itself like the blade of a jack- or clasp-knife. The reflex is thought to be mediated by A-b and A-d sensory neurons with free nerve ending receptors located in muscle fascia and aponeuroses. The spinal circuits responsible for the clasp-knife reflex are normally suppressed and only become operational after lesions to the spinal cord or brain.