G Protein Coupled Receptors
Mark Bothwell
Introduction: What is Cellular Signaling?
Signaling means communication of information. Familiar biological examples include actions of endocrine hormones and neurotransmitters.
Cellular signaling requires at least four steps.
- creation or release of a chemical as a message,
- binding of the message molecule to a receptor protein at the target,
- initiation of a response at the target, and
- removal of the message.
Often the response in the target cell is an intracellular message, sometimes called a second messenger, that is recognized by another internal receptor and initiates another response. Ultimately a cascade of such intracellular events may produce several biological outputs. Examples of intracellular second messengers are rises of cyclic AMP or of Ca2+, and examples of responses are speeding of heart rate or inducing gene expression. Over two hours class hours we will discuss three classes of receptors: the G protein coupled receptors, the tyrosine kinase receptors, and the nuclear receptors.
This chapter focuses on G protein-coupled receptors (GPCRs), which represent the largest family of plasma membrane receptors. The human genome contains over 800 GPCR genes. Among the ligands for GPCRs are monoamine neurotransmitters (adrenaline, noradrenaline, serotonin, dopamine, histamine), many peptide hormones, light photons, odorants, and sweet, bitter, and “umami” tastes (Box A). For many of these extracellular stimuli there are multiple different GPCRs, meaning that the same extracellular signal might give rise to different intracellular responses depending on which receptor subtype it encounters. For example, there are three major types of receptors for adrenaline and noradrenaline, called α1, α2, and β adrenergic receptors. In turn, each receptor type is further diversified by being encoded by three slightly different genes, making a total of nine adrenergic receptor genes altogether. The GPCR family is exceptionally important clinically, as demonstrated by the fact that 35% of FDA-approved drugs target GPCRs or elements of their down-stream signaling pathways.
Box A: Diversity of GPCR ligands
Neurotransmitters
- norepinephrine
- adenosine
- histamine
- serotonin
- dopamine
- cannabinoids
- opiates
- acetylcholine
- GABA
- glutamate
Peptide hormones
- endothelin
- glucagon
- angiotensin II
- GSH, FSH, CRH
- GnRH, ACTH
Tastants, oderants, light
Learning Objectives
1. Describe in detail the activation of GPCR signaling including receptors, ligands & heterotrimeric G-proteins.
2. Know different G-proteins coupling to different second messengers.
3. Describe two effector pathways: cAMP and PLC.
4. Know how GPCR signaling is terminated.
GPCR Structure and Function.
GPCRs are integral plasma membrane proteins with 7 transmembrane segments (Figure 1). GPCRs transduce signals from extracellular ligands to signals in intracellular relay proteins, the hetero-trimeric GTP binding proteins (G proteins). By coupling to many downstream intracellular cascades and effectors, the G proteins initiate pleiotropic changes in many intracellular targets.
Ligand -> GPCR -> G protein -> 2nd messengers -> many intracellular effectors
Thus, extracellular signals are typically amplified to produce robust, varied, and cell-specific intracellular responses.
Signaling from GPCRs to G proteins.
GPCRs couple to intracellular, GTP-binding, heterotrimeric G proteins that consist of Gα, Gβ, and Gγ subunits (Figure 1). At rest these three G protein subunits are assembled into the heterotrimeric complex, Gαβγ. Since Gβ and Gγ are inseparable once co-assembled, it is customary to talk about a resting complex of Gα with Gβγ. Gα and Gβγ are not transmembrane proteins but they are tethered at the inner leaflet of the plasma membrane by hydrophobic lipid modifications.
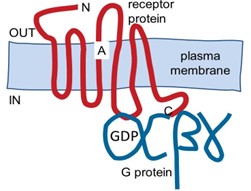
Gα subunits are powerful signaling proteins. In the inactive resting Gαβγ complex, the Gα subunit is bound to the guanine nucleotide, guanosine diphosphate (GDP), but when a GPCR is activated, the receptor can catalyze a ”nucleotide exchange” reaction” on the Gα subunit (Figure 2).
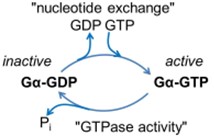
GDP leaves and guanosine triphosphate (GTP) binds instead. GTP binding activates the G protein (hence the name). GTP is a high-energy molecule similar to ATP except that is formed from guanosine rather than adenosine. Once formed, the Gα-GTP-Gβγ complex is unstable so that the active Gα-GTP and Gβγ separate from one another and from the receptor as well (Figure 3).
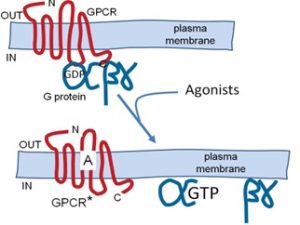
However, the G protein subunits normally still remain attached to the plasma membrane by their lipid anchors. Thus there are now three active products that couple to downstream effectors: the activated receptor, the Gα-GTP subunit, and the Gβγ dimer. The activated receptor is again free to couple repeatedly with additional G proteins, amplifying the signal. Because the activated G proteins are membrane associated, the next step will be an interaction with membrane-associated effector proteins or recruitment of cytoplasmic effector proteins to the membrane. Gα is both a regulatory switch and an enzyme. It slowly cleaves the bound GTP to give GDP (Fig. 2). Gα-GDP is inactive, binds Gβγ again, and waits for a new round of activation. This exemplifies a typical role of GTP in biology–as a timer of switches and as an informational molecule.
Five families of Gα-GTP subunits.
As Gα-GTP and Gβγ each has the capacity to convey cellular signals we will discuss them separately, focusing primarily on Gα-GTP.
Since there are hundreds of types of GPCRs, one might expect hundreds of types of downstream signals. However, that is not the case. There are only five families of G protein α-subunits, giving five kinds of responses. Although we name the five G protein families below for completeness, we will focus discussion on only two of them (Gs and Gq). At least 50 types of GPCRs are specialized to signal through each of the first 4 families.
- Gαs (s for “stimulatory”) was the first G protein discovered. It stimulates plasma membrane adenylyl cyclases, increasing cellular cyclic AMP (cAMP), which, e.g., stimulates phosphorylation of target proteins by cAMP-dependent protein kinase. Gαs is the target of cholera toxin, which activates Gαs and its downstream signaling. In the gut, this irreversible activation is the signal inducing diarrhea in cholera.
- Gαi/o (i for “inhibitory” and o for “other”) G proteins inhibit most adenylyl cyclases, decreasing cellular cAMP responses. Gαi/o is the target of Pertussis toxin, which turns them off. Gαo is said to constitute 1% of brain proteins. Being so abundant, it is a major source for active Gβγ subunits.
- Gαq activates phospholipase Cβ (PLCβ), a lipase enzyme that cleaves the signaling phosphoinositide lipids (PIP2) of the plasma membrane, generating several key 2nd messengers IP3 and diacylglycerol. We expand on these signals in a moment.
- Gα12 enhances RhoA, Rho kinase, changes expression of some genes, and slows dephosphorylation of myosin light chains affecting smooth muscle tone.
- Gαtransducin is found only in retinal photoreceptors. Transducin transduces the light response from rhodopsin by activating cyclic GMP (cGMP) phosphodiesterase that cleaves and depletes cytoplasmic cGMP. In vision, each photon depletes a small fraction of photoreceptor cGMP, generating a small electrical signal in cGMP-gated ion channels.
Each of these pathways involves second messengers and effector enzymes. The long cascade of signaling may take up to tens of seconds to be completed. However in a few cases, such as vision using rhodopsin and transducin, an extreme high density of the receptors and G proteins and miniaturization of the geometry have allowed responses that take only tens of milliseconds. When we watch the world, vision through rhodopsin and Gαtransducin, is so fast that we are not aware of any time delay. On the other hand, increases of heart rate during exercise, mediated by Gαs and cAMP, take minutes.
Downstream coupling of Gβγ
The Gβγ subunits are potent plasma membrane signals as well. They act on several ion channels in the membrane and on several signaling enzymes, but they will concern you only later and are not detailed here.
Signaling from Gαs to cAMP
As Figure 4 summarizes, the pathway from Gs is a long signaling cascade. Since there are at least three stages of amplification (catalysis by the GPCR and catalytic enzymatic activities of two enzymes), the signal from even a single molecule of ligand is greatly amplified and the response can be robust. Every cell in the body has specialized responses to cAMP signaling. Gαs, or Gs as it is called for short, activates the membrane enzyme adenylyl cyclase, which converts ATP into cytoplasmic 3′,5′-cyclic adenosine monophosphate (cAMP). This diffusible intracellular 2nd messenger in turn is an activating ligand for the cytoplasmic enzyme, cAMP-dependent protein kinase (PKA). As its name implies, PKA uses ATP to phosphorylate specific cellular proteins (on the hydroxyl groups of serine and threonine). This is a regulatory step. The number of target proteins regulated by PKA phosphorylation is large—dozens in a single cell. Three prominent examples include, (i) phosphorylation and recruitment of voltage-gated Ca2+ channels in heart to increase contractility, (ii) phosphorylation of phosphorylase kinase, which in turn phosphorylates phosphorylase, which releases glucose-1-phosphate from glycogen and boosts energy metabolism in liver, and (iii) response to odorants by sensory neurons in the olfactory epithelium by direct action of cAMP on opening of cAMP-gated ion channels of the plasma membrane of olfactory neurons.
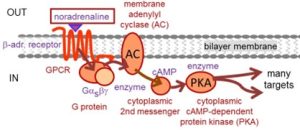
Signaling from Gαq to PLC
Gq elicits very different responses that Gs. The Gq signaling branches at once into two pathways, shown as blue and green pathways in Fig. 5. Gq-GTP recruits the cytoplasmic enzyme phospho-lipase C (PLC) to the membrane where it cleaves the polar head group from the membrane phospholipid phosphatidylinosiitol 4,5-bisphosphate (PIP2). This yields (i) the membrane lipid component, diacylglycerol (DAG), and (ii) the soluble cytoplasmic component, inositol 1,4,5-tris phosphate (IP3). Each is a key 2nd messenger. In the blue pathway, DAG recruits and activates the cytoplasmic enzyme protein kinase C (PKC), and PKC phosphorylates specific target proteins (again on hydroxyls of serine and threonine). The end results are analogous to phosphorylations by PKA, but the targets and responses are not identical. In the green pathway, the diffusible intracellular IP3 messenger is a ligand for a Ca2+-permeable ion channel, the IP3 receptor (IP3R), on the endoplasmic reticulum (ER) (Figure 5). When the channel opens, Ca2+ flows from the ER to the cytoplasm. The resulting Ca2+ rise triggers many Ca2+-sensitive cellular processes, such as, secretion, contraction, or altered gene expression.
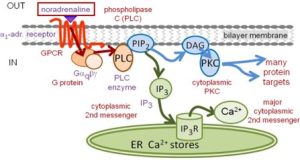
Termination of GPCR signaling
Termination of signaling requires turning off activated receptors, turning off activated G-proteins, and return of second messenger levels, protein phosphorylation levels, and other changed metabolites to their original values. Consider the first two. Receptors quickly deactivate upon unbinding and washing away of the agonist ligand. They are also inactivated by other processes even while ligand is still present. Such mechanisms that prevent over-stimulation are usually called receptor desensitization. In one canonical shutdown pathway, activated receptors are recognized and phosphorylated by G-protein coupled receptor kinases (GRKs) (Figure 6). Phosphorylated receptors may have reduced activity, and they can be turned off fully by binding of arrestins at the plasma membrane. The arrestin-receptor complex may be unable to couple to downstream G proteins, but it can mediate other signaling and it also may be removed from the plasma membrane by clathrin-mediated endocytosis, a true down regulation of receptor protein. A loss of function mutation in the GRK1 gene leads to stationary night blindness Oguchi type-2 where a severe retardation of dark adaptation of rhodopsin (the GPCR) in rod photoreceptors impairs vision at low light levels.
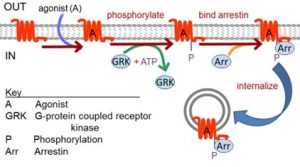
As we mentioned already, signaling by activated Gα-GTP is terminated by GTP hydrolysis, a reaction catalyzed by the Gα subunit itself that yields the inactive form, Gα-GDP (Figure 2). Thus activated G-proteins have an intrinsic self-timer function that terminates their activity. The resulting Gα-GDP in turn is a scavenger that binds any free Gβγ dimers, re-forming the inactive heterotrimeric G protein GαβγGDP.
Note: The heterotrimeric G proteins discussed here should be distinguished from the small, low molecular weight, monomeric G proteins of the Ras, Rab, Rho, etc. families with which the Gα subunit does share some homology (Box B).
Box B: Heterotrimeric versus monomeric G proteins
Heterotrimeric G proteins
- Have three subunits: αβγ
- Activated by GPCRs
- Active when GTP is bound to α
- Turned off when α cleaves GTP to GDP
- The α subunit has much homology with the monomeric G proteins
Monomeric G proteins
- Have only one subunit
- Low molecular weight
- Active when GTP is bound
- Turned off when they cleave GTP to GDP
Signaling by Ras will be discussed in the section on signaling by receptor tyrosine kinases. The monomeric G proteins have the same GTP-binding and GTPase properties as Gα subunits of heterotrimeric G proteins (Figure 2), and they both use the bound GTP to time their active signaling life time. But the monomeric G proteins do not couple to GPCRs or to Gβγ, and they signal to quite different downstream effectors.
Test your knowledge
Feedback:
G proteins are so-named because they bind GDP or GTP. They signal actively when GTP is bound and signaling terminates when their intrinsic GTPase converts GTP to GDP. "Heterotrimeric" refers to their makeup of three different subunits; the GTP/GDP-binding alpha subunit, and the modulatory beta and gamma subunits.
a G protein-coupled receptor that mediates vision in dim light by controlling activity of transducin, a G protein
an enzyme that catalyzes the conversion of ATP into cytoplasmic 3',5'-cyclic adenosine monophosphate (cAMP)
an ion channel that allows transmembrane passage of calcium ions in a manner that is regulated the membrane voltage gradient
proteins that bind G protein-coupled receptors to inhibit signaling through G protein activation and ultimately, to permanently terminate signaling by promoting endocytotic internalization and degradation of the receptor