2 Brain-Computer Interfaces (BCI)
Welcome to the second day of the YSP-REACH Program! Today we will explore how and why researchers are working to connect the human brain with computers and machines. These neurotechnologies are known as brain-computer interfaces (BCIs) or brain-machine interfaces (BMIs). In today’s session, Dr. Rajesh Rao (a faculty member in the UW Department of Computer Science and Engineering and the co-Director of the Center for Neurotechnology) will share his cutting-edge research.
Stop for a minute and think to yourself:
- Why would we want to connect a brain to a computer or a machine?
- What might be the benefits?
- What might be the challenges, problems, or unintended consequences when we connect the human brain with technology?
Try It!
Respond to this question on a Padlet:
- What might be the challenges, problems, or unintended consequences when we connect the human brain with technology?
Post a brief response to this Considerations for BCI Technology Padlet. See what other YSP-REACH participants have posted as well. You can “favorite” the ones you agree with or add comments.
Then, hit the “back” arrow on your web browser to return to this chapter and keep reading.
Part 1: What is a Brain-Computer Interface?
We will launch today’s exploration of brain-computer interfaces with a video from the BrainWorks series from UW TV in which Dr. Eric Chudler and a group of teens explore how BCIs work. This video is 27 minutes long, so grab a cup of tea and hit play. English captions are available.
Reflect on What You Learned
After watching the video, check your knowledge with a short quiz.
How do BCIs work?
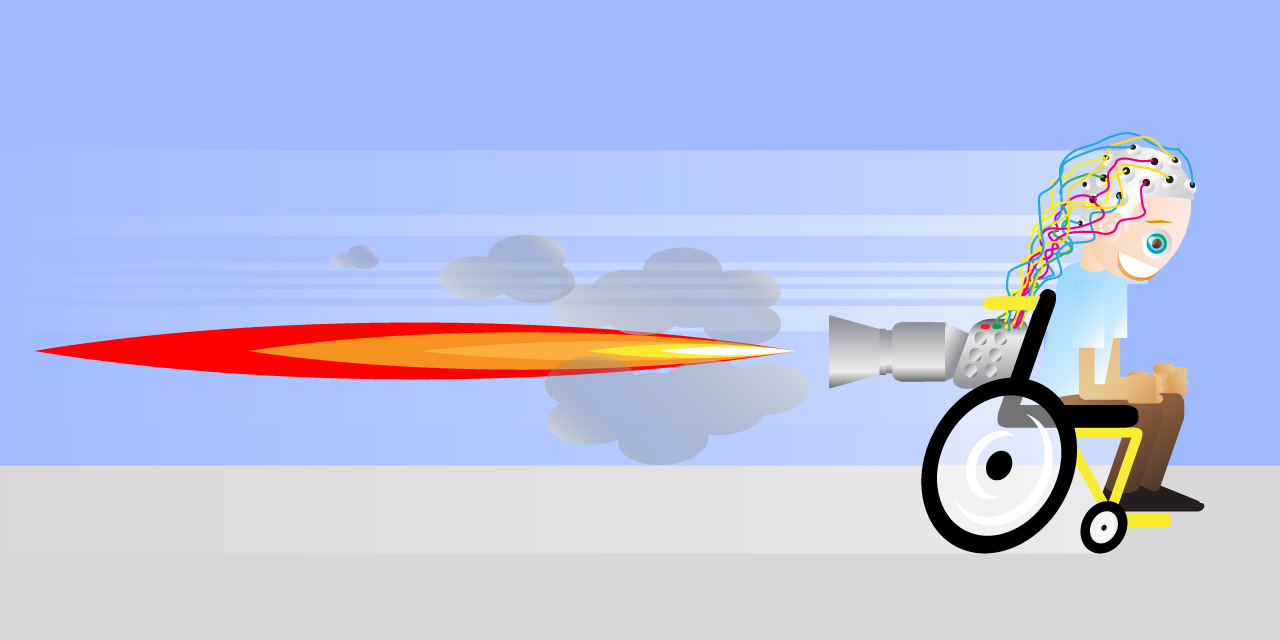
Let’s dig in a little deeper to understand how BCIs work. Read the article, “Brain-Machine Interfaces: Your Brain in Action”, which provides an overview of how BCIs/BMIs work, and in particular, how they use biosignals generated by the body to communicate with computers and machines.
Read This!
Read the article: “Brain-Machine Interfaces: Your Brain in Action.” As you read, consider the three major challenges of BCIs/BMIs that are described in the article.
Citation: Carmena J and Millán J. (2013). Brain–Machine Interfaces: Your Brain in Action. Front. Young Minds. 1:7. doi: 10.3389/frym.2013.00007
Reflect on What You Learned
After reading the article, complete a short quiz. Re-arrange the images below to show the process of how a BCI or BMI works. Click on the text below each picture to read the entire clue.
Part 2: Biosignals
As the video and article pointed out, biosignals are an important component of BCIs. Biosignals are signals generated by the human body that can be measured. As you learned yesterday, neurons produce both electrical and chemical signals in order to communicate. Bioelectrical signals are electrical signals produced by the nervous system (e.g., EMG, EEG, ECoG) or organ systems (e.g., ECG/EKG, GSR). Electrical signals produced by action potentials in the nervous system are of major interest to neural engineers. Many neuroprosthetics communicate with the human body through the use of EEG or ECoG electrodes that record neural signals from the brain or spinal cord. Some neuroprosthetic devices rely on EMG electrodes that record skeletal muscle activity, or more precise electrodes (intraneural and extraneural) that can record from individual neurons in the peripheral nervous system. Some devices, including deep brain simulators (DBS) use electrodes surgically inserted into the brain. Here is a quick overview of ways of measuring and recording these biosignals:
- ECoG (Electrocorticography): Monitors brain signals with electrodes that are placed on the surface of the brain. Invasive, requires surgery.
- EEG (Electroencephalography): Monitors brain activity with electrodes that are placed on top of the scalp. Non-invasive.
- EMG (Electromyography): Monitors skeletal muscle activity with electrodes that are placed on the skin. Non-invasive.
- Intracerebral: Directly records activity from neurons with electrodes that are surgically inserted into brain tissue. Invasive, requires surgery.
Neuroprosthetics are able to communicate with the human body by using electrodes that record bioelectrical signals generated by the brain, spinal cord, skeletal muscles, and peripheral nerves.
Look at This!
Check out this amazing graphic on “Brain Interfacing” to learn about the pros’ and con’s of each of these ways of recording and measuring neural signals. (Credit for this graphic goes to Scott Leighton.) Then hit the “back” arrow on your web browser to return to this chapter and continue reading.
Neural engineers are able to obtain these biosignals by using electrodes to measure and record the signals. Electrodes are conductors in which electricity enters or leaves an object. In neuroscience, electrodes are used to detect biosignals produced by the body. Electrodes are used in EEG, EMG, ECoG, and other methods to record the electrical activity generated by neurons. These electrode can be placed on the surface of the skin, onto the surface of the brain under the skull, or inserted directly into the brain or spinal cord. Some electrodes are engineered to also be able to provide electrical stimulation, light (optogenetic) stimulation, and/or deliver small amounts of pharmaceutical drugs. These kinds of electrodes are made from metal, glass, glassy carbon, and other materials that do not react when surgically implanted into the body. Some electrodes are made to be flexible so that they can move with the tissue.
Optional: Read and Watch to Learn More!
If you would like to learn more about biosignals, the article “Neuroprosthetics” from The Scientist and the video “Unleashing the Mind: The Future of Brain-Computer Interfaces and Neural Implants” provides a deeper explanation of how neuroprosthetics use electrodes to measure and record biosignals.
Open-Loop vs. Closed-Loop BCIs
In an open-loop BCI, the person using the device does not receive any feedback about the operation of the system. However, in a closed-loop BCI, the users see, hear or feel what the device is doing and can use this information to change what they are doing. It may even be possible to develop a BCI that sends sensory feedback via electrical stimulation of the brain.
Part 3: CNT Research Spotlight
Researchers at the Center for Neurotechnology are involved in many aspects of developing better brain-computer interfaces, including cochlear implants, deep brain stimulation, brain-to-brain communication, and prosthetic limbs. In today’s CNT Research Spotlight, we focus on a team of researchers that are using closed-loop BCI technology to develop prosthetic limbs that allow the users to touch and feel. First, listen to a radio story and then read a short article about these amazing neuroprosthetics.
Listen to This!
Listen to a short three-minute radio story from KNKX about Dr. David Caldwell, a bioengineer at the University of Washington. “UW Team Works to Develop Prosthetic Limbs that Help Users Touch and Feel.”
(A transcript is not available, but there is a brief article covering similar information on the website.)
Read This!
After listening to the radio story, read the article, “An Unexpected Difference in Reaction Times to Artificial and Natural Touch” to learn more about Dr. Caldwell’s research project.
Part 4: Types of Brain-computer interfaces
There are three common types of brain-computer interfaces. These include:
- Neuroprosthetics
- Retinal Implants
- Cochlear Implants
Optional: Learn More!
If you would like to learn more about BCIs, we’ve curated a list of learning resources for you to explore, organized by type of BCI. Enjoy!
Option 1: Learn More About Neuroprosthetics (Bionic/Robotic Limbs)
Learn more about research breakthroughs in the field of neuroprosthetic limbs by exploring these resources:
- Want to learn more about Dr. Caldwell’s incredible prosthetic limbs? Check out the graphics and skim the text in the research article “Direct Stimulation of Somatosensory Cortex Results in Slower Reaction Times Compared to Peripheral Touch in Humans,” published in 2019 in Nature. Reading scientific journal articles can be tough, so be sure to review our resource on How to Read an Article from an Academic Journal for helpful tips.
- “Smart Bionic Limbs are Reengineering the Human” describes the machine intelligence approach being used by scientists to create bionic limbs. This article was published in 2012 in Popular Mechanics.
- A brief article about smart prosthetic arms, “Neuroprosthetics: Once More, With Feeling” is available from the journal, Nature.
Option 2: Learn About Cochlear Implants (for deafness and hearing impairments)
Learn about cochlear implants by exploring these resources. You will need speakers or headphones for many of these online activities.
- The article, “Bionic Senses” from Harvard University, provides background information about how neuroprosthetics can help restore sight and hearing through the use of cochlear and retinal implants.
- This factsheet from the NIH provides a good introduction to cochlear implants.
- What is it like to experience hearing loss? Try out the Hearing Loss Simulator from Hearing Like Me to help you understand mild and moderate hearing loss. Listen to sixteen different recordings at normal hearing compared to mild and moderate hearing loss.
- Hear the World Foundation also has an online demonstration of What Hearing Loss Sounds Like. You will need speakers or headphones for this to work.
- What is it like to have a cochlear implant? The Cochlear Implant Audio Demos (developed by Philipos C. Loizou) allows you to explore what happens when the number of channels is changed or the depth of the electrode is adjusted on the clarity of human speech processed through a cochlear implant.
- It is important to note that cochlear implants can be controversial, because some people believe that cochlear implants are an affront to the Deaf community and their culture.
Option 3: Learn about Retinal Implants (for blindness and vision loss)
Learn about retinal implants by exploring these resources:
- The Scientist provides a series of short articles that offer a general overview of retinal implant devices.
- The article, “Bionic Senses” from Harvard University, provides background information about how neuroprosthetics can help restore sight and hearing through the use of cochlear and retinal implants.
Tomorrow we will focus on treatments for stroke. Our guest speaker will be Dr. Azadeh Yazdan-Shahmorad, who is developing neurotechnological solutions for patients who have suffered from a stroke.
Optional Resources
- Learn about how BCIs are being used more broadly by reading the 2019 article “Mind-reading systems: Seven Ways Brain Computer Interfaces are Already Changing the World“.
- Want to learn more about Dr. Caldwell’s incredible prosthetic limbs? Check out the graphics and skim the text in the research article “Direct Stimulation of Somatosensory Cortex Results in Slower Reaction Times Compared to Peripheral Touch in Humans,” published in 2019 in Nature. Reading scientific journal articles can be challenging, so be sure to review our resource on How to Read an Article from an Academic Journal for helpful tips.
- Brown University’s BrainGate channel on YouTube (www.youtube.com/playlist?list=PL789FA64D163DF6D0) features videos that show patients controlling external robotic arms using BCI technology.
Citations:
- BCI and wheelchair image: Carmena J and Millán J. (2013). Brain–Machine Interfaces: Your Brain in Action. Front. Young Minds. 1:7. doi: 10.3389/frym.2013.00007.
- Icons for quiz: Flaticon.com.
- Research highlight photos: Center for Neurotechnology.
A communication pathway linking neural signals from the brain with an external device. The neural signals are decoded by a computer using an algorithm that translates the signals into a motor output (action), such as controlling a neuroprosthetic hand or steering a wheelchair. BCIs may operate using either EEG or ECoG recordings or single unit activity from neurons. Also called a Brain-Machine Interface or a Brain-Control Interface.
A signal generated by the human body that can be measured. Bioelectrical signals are electrical signals produced by the nervous system (e.g., EMG, EEG, ECoG) or organ systems (e.g., ECG/EKG, GSR).
The nervous system’s communication method is via action potentials, which are electrochemical changes that originate in neurons, are propagated down their axons, and elicit a corresponding response in the adjacent neurons.
An engineered device that interfaces with the nervous system to support abilities lost due to damage or disease or to enhance existing body function.
A conductor in which electricity enters or leaves an object. In neuroscience, electrodes are used to detect biosignals produced by the body. Electrodes are used in EEG, EMG, ECoG, and signal units to record the electrical activity within neurons.They may be laid on the surface of the skin, onto the surface of the brain under the skull, or inserted directly into brain tissue or the spinal cord. Some electrodes are being engineered to also be able to provide electrical stimulation, light (optigenetic) stimulation, and/or deliver small amounts of pharmaceutical drugs. These kinds of electrodes are made from metals, glass, glassy carbon, and other materials that do not react when surgically implanted in an animal’s body. Some are made to be flexible so that they can move with the spinal cord.
A technique for recording the electrical activity of the brain obtained from electrodes placed on the surface of the brain. ECoG is an invasive way to record signals from the brain, requiring surgery to access the brain’s surface.
A technique for recording electrical activity of the brain obtained from electrodes placed on the scalp. EEG is a non-invasive way to record brain signals.
A technique for recording the electrical activity of skeletal muscles obtained from electrodes placed on the skin. EMG is a non-invasive way to record motor neuron activity.
A type of electrode that is able to directly records neurons with incredible precision. These special electrodes are inserted into brain tissue through an invasive surgical procedure.
A cochlear implant is an electronic device created for people, and most often children, with a certain kind of significant hearing loss known as sensorineural hearing loss. A cochlear implant bypasses damaged hair cells in a part of the inner ear known as the cochlea. The device consists of an implanted (internal) receiver and electrodes, and an external microphone, speech processor and transmitter.
A prosthetic device surgically implanted in the eye to restore some vision to people with certain types of vision loss. The device consists of the implant, and special eyeglasses that contain a camera and transmitter. The implant consists of an array of tiny electrodes that electrically stimulate inner retinal neurons.