Autonomic Nervous System Physiology
John Tuthill
Learning Objectives and Quick Synopses
1. Compare and contrast the neurotransmitters and receptor types in the somatic motor division, parasympathetic autonomic efferent division, and sympathetic autonomic efferent division of the nervous system. Include the neurotransmitter / receptor pairs in the ANS two-neuron pathways.
Preganglionic nerve fibers exit the central nervous system (CNS) and release acetylcholine (ACh) on postganglionic neurons in autonomic ganglion. This second postganglionic neuron releases norepinephrine on targets if it is sympathetic, or ACh if it is parasympathetic. The ACh receptors on the ganglion are nicotinic type, whereas the distal receptors are muscarinic type on parasympathetic target cells and adrenergic on sympathetic target cells.
2. Identify epinephrine / norepinephrine receptor types and their effects on various target organs.
There are two categories of alpha adrenergic receptors and three types of beta adrenergic receptors. They are G-protein coupled receptors (GPCRs). You will learn the G proteins they each couple to and some principal cell types they are expressed in (see Tables).
3. Compare nicotinic and muscarinic acetylcholine receptor (AChR) activation and identify acetylcholine receptor types and their effects on various target organs
Nicotinic nAChRs are fast, ligand-gated nonselective cation channels. Muscarinic mAChRs are GPCRs and not ion channels. You will learn the G proteins that M2 and M3 muscarinic receptors couple to and some principal cell types they are expressed in (see Tables).
4. Identify the role of ATP and nitric oxide in smooth muscle relaxation and blood vessel-dilation.
ATP is released by exocytosis from synaptic vesicles as a cotransmitter from autonomic nerve fibers. It can initiate contraction of smooth muscles in arterioles and in the vas deferens. The diffusible gas nitric oxide (NO) is synthesized enzymatically in neurons of the genitalia and in endothelium of the cardiac coronary circulation. It relaxes smooth muscle, stimulates blood flow, and signals erection.
5. Describe the baroceptor reflex in response to high or low blood pressure.
The baroceptor reflex is an autonomic reflex that excites the parasympathetic NS and inhibits the sympathetic NS when blood pressure rises. The reflex path is: Pressure receptor -> CNS -> ANS.
6. Define orthostatic hypotension and discuss how the baroreflex counters it in healthy patients.
Orthostatic hypotension is a loss of blood pressure in the upper body that happens when you stand up. Pressure receptors mount a corrective response called the baroreflex.
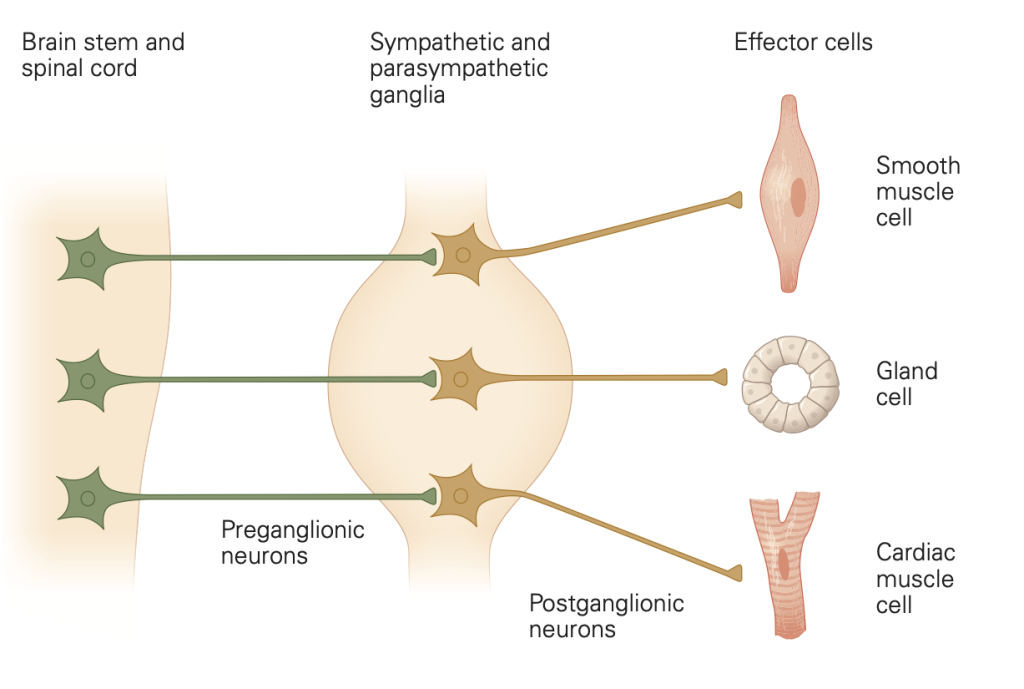
The word efferent means “carrying messages away from the central nervous system (CNS).” The CNS communicates to the rest of the body through three major classes of efferent nerve fibers: (i) somatic, (ii) sympathetic preganglionic, and (iii) parasympathetic preganglionic. The first controls familiar voluntary muscle motor activity. The latter two belong to the autonomic nervous system (or simply, ANS), the subject of this chapter. The word autonomic implies involuntary, happening by itself. The agenda of these three efferent systems is different, crudely: (i) voluntary movement, (ii) fight & flight, and (iii) rest & These three classes of efferent nerve fibers have their cell bodies in the spinal cord or brain stem and send axons out as nerve bundles to the periphery. Such efferent nerve fibers all act by releasing the neurotransmitter acetylcholine (ACh) on their initial targets, so by definition they are called cholinergic (colored blue here). Here we focus on the neurotransmitters, receptors, and specialized cellular effector actions of ANS signaling.
(Unless otherwise noted, all figures are from: Kandel ER, Schwartz JH, Jessell TM 2012, Siegelbaum SA, Hudspeth AJ. ‘Principles of Neural Science, 5th ed. McGraw-Hill, New York.)
Somatic nervous system efferents
Efferent somatic motoneurons innervate skeletal muscle forming a very large synapse: the neuromuscular junction (NMJ) or motor endplate. They excite action potentials in the muscle by opening depolariz- ing nicotinic ACh receptor ion channels (nAChRs) at the NMJ. Each skeletal muscle fiber is an obligatory follower of the motor axon that innervates it. Each presynaptic action potential propagating in a somatic motor axon elicits one postsynaptic action potential in the skeletal muscle fibers it innervates and one muscle twitch.
Autonomic nervous system efferents
Almost every organ of the body is innervated by the two autonomic peripheral systems called the sympathetic and parasympathetic nervous systems. In both, the innervation is via a two-neuron relay: first efferent preganglionic neurons and then peripheral postganglionic neurons. The efferent ANS nerve fibers that exit the CNS are called preganglionic because they release their ACh in the peripheral autonomic ganglia to excite the second postganglionic neurons that in turn innervate the peripheral targets of the ANS. The ANS efferent preganglionic nerve fibers form standard, focal, and fast nicotinic synapses on ANS postganglionic neurons. As it is for skeletal muscle, the ACh effect within the ganglion is depolarizing and excitatory, and the postganglionic neuron fires action potentials, acting mostly as a follower: Each preganglionic action potential elicits one or a few postganglionic action potentials in the second neuron. Thus, the somatic motor efferents and the ANS efferents share a common neurotransmitter (ACh) and act on quite similar (but genetically distinct) nicotinic receptors.
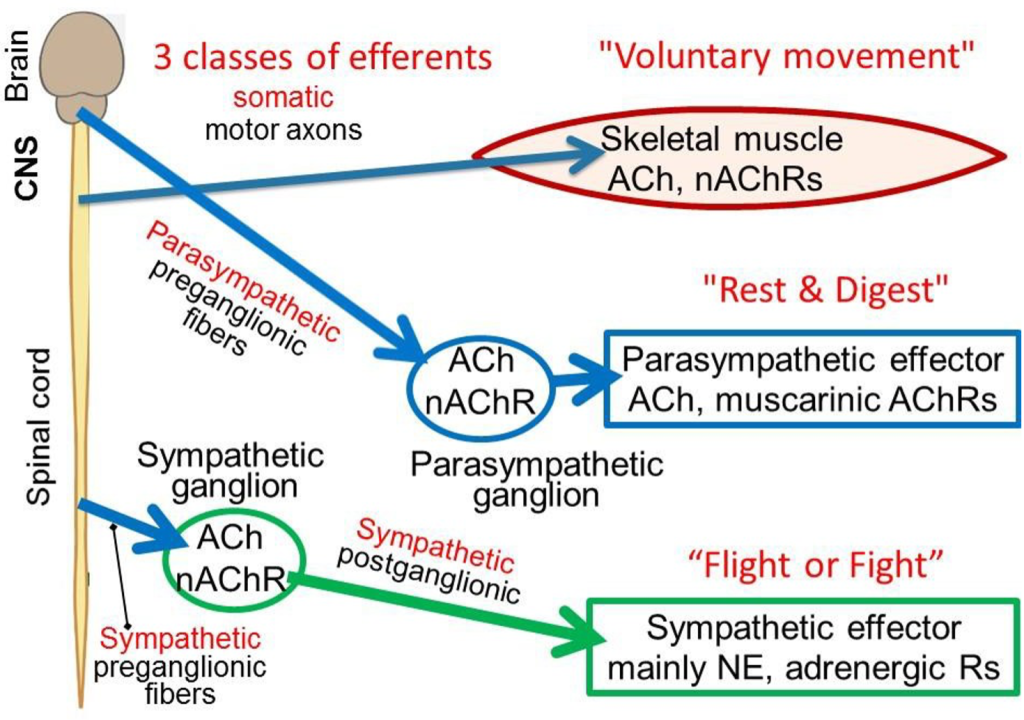
Autonomic postganglionic nerve fibers
We have emphasized that autonomic efferent signals are relayed in a second step by axons of post-ganglionic neurons to their final targets in a two-neuron efferent pathway (Figure 2)–we call this the “two-neuron rule.” The targets include glands, all muscles, the major organs, epithelia, and blood vessels. Instead of forming terminal focal synapses with point-to-point connections, the postganglionic neurons (the second neurons) of the ANS usually deliver neurotransmitters more diffusely throughout a target tissue by “sprinkled” release from numerous varicosities along the length of their axons. The varicosities are swellings–looking like beads on a string–that you could think of as sprinkler heads arranged along a water pipe. As is true of fast, focal synapses, the varicosities release their neurotransmitters in response to passing action potentials by calcium-dependent exocytosis from intracellular neurotransmitter vesicles concentrated in the varicosity. You should think of the actions of autonomic nervous system axons as broadly regulating groups of cells and tissues rather than as targeting a specific single cell.
An exception to the two-neuron rule
There is an exception to the two-neuron rule in the adrenal gland. The epinephrine-secreting chromaffin cells of the adrenal medulla are directly innervated by the “preganglionic” cholinergic efferent nerve rather than by a two-neuron pathway. However, loosely, one might regard the neuron-related, specialized secretory chromaffin cells as being like postganglionic neurons that deliver their epinephrine hormone/neurotransmitter by vesicular exocytosis, but now in endocrine fashion. They are round cells with no axons but release the hormone from the cell body to blood that circulates everywhere.
Sympathetic versus parasympathetic postganglionic neurotransmitter
We have emphasized the common features of postganglionic neurons. We now concentrate on the specialized properties that differentiate the postganglionic sympathetic from the postganglionic parasympathetic nerve fibers–with reference again to Figure 2.
• Sympathetic postganglionic nerve fibers are adrenergic. They release norepinephrine (NE) by exocytosis from synaptic vesicles in their varicosities. (Colored green in Figure 2)
• Parasympathetic postganglionic fibers are cholinergic. They release ACh by exocytosis from synaptic vesicles in their varicosities. (Colored blue in Figure 2.)
• Both classes of postganglionic nerve fibers release additional mediators, sometimes called cotransmitters, in addition to NE or ACh. This may include neuropeptides and other small molecules such as ATP or nitric oxide. We do consider them later, but here we emphasize the principal neurotransmitters NE and ACh and their actions.
• Exception: At eccrine sweat glands, sympathetic postganglionic fibers release ACh instead of NE.
Receptors at each synapse
Nicotinic ACh receptors (nAChRs) are fast ligand-gated ion channels used at the cholinergic synapses on skeletal muscle endplates and at the synapses on all postganglionic neurons in autonomic ganglia. They are depolarizing (excitatory), nonselective cation channels that initiate action potentials. These nAChRs relay electrical excitation (action potentials) from the presynaptic neuron to a postsynaptic cell.
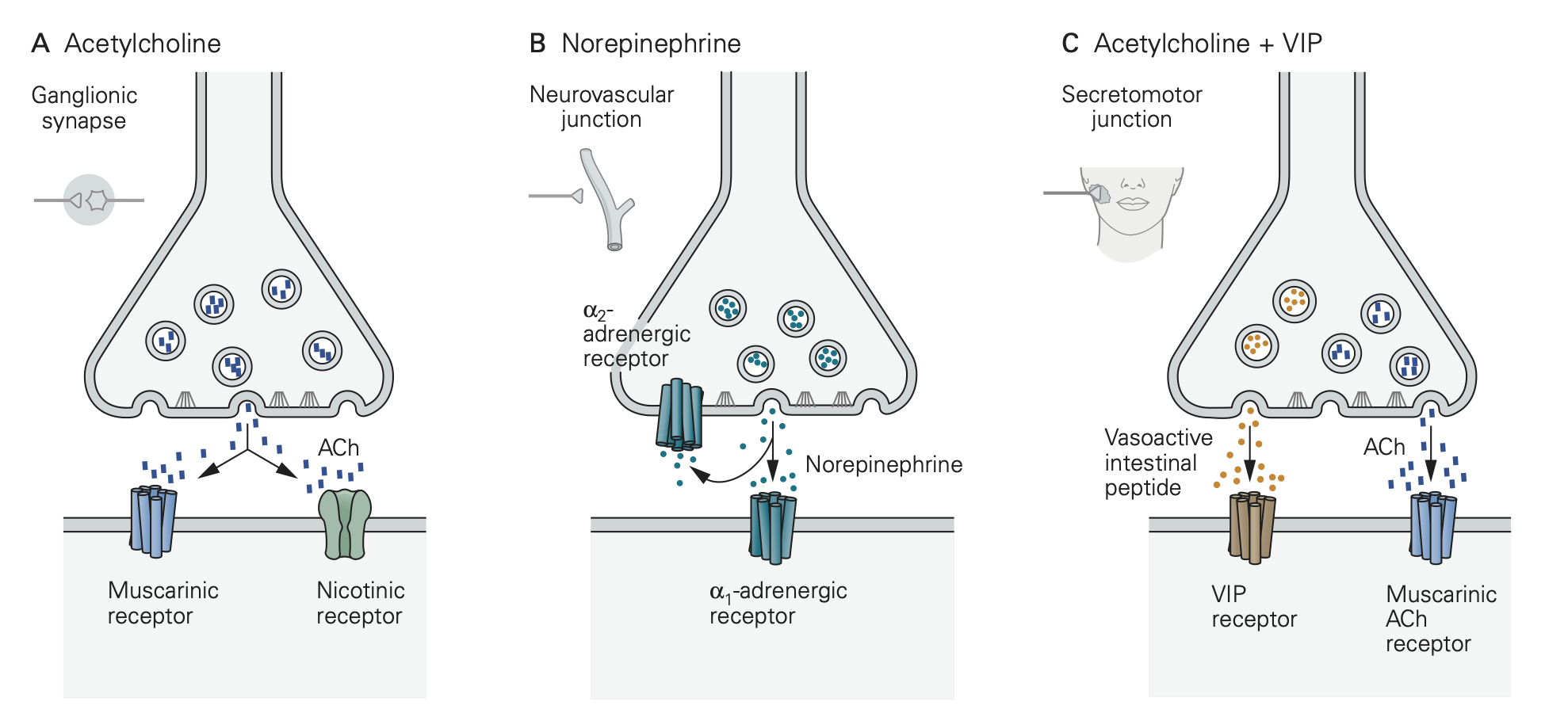
Thus, in the ganglion, they help to relay preganglionic action potential messages as postganglonic action potential messages on their way to target organs.
By contrast, various G-protein coupled receptors (GPCRs) are the principal receptors for neurotransmitter in the target organs of the postganglionic sympathetic and parasympathetic nerve fibers. Recall that GPCRs are not ion channels. Rather they initiate cascades of G-protein, calcium, and protein kinase signaling. Thus, action potentials and transmitter release from the postganglionic nerve fibers initiate biochemical signaling cascades in the target tissues, rather than action potentials. Like the postganglionic varicosities that stimulate them, these GPCRs on the target are not discretely organized in specialized postsynaptic structures but rather are diffusely spread over the surface of target cells and tissues. For ANS responses the key GPCRs are:
- Muscarinic ACh receptors (mAChRs, several kinds) mediating parasympathetic effects.
- Adrenergic receptors (α1, α2, and β) mediating sympathetic effects.
- Additional receptors responding to other cotransmitters like ATP, peptides, and nitric oxide (NO).
Table 1: Neurotransmitters and receptors of the ANS.
Learning Objective #2: Identify epinephrine / norepinephrine receptor types and their effects on various target organs.
Norepinephrine, Epinephrine, and their Adrenergic receptors
Norpinephrine (NE) and epinephrine (E) are the principal sympathetic neurotransmitters.
- NE is released locally on tissue targets by most sympathetic postganglionic nerve fibers at their varicosities. The released NE is taken up again into the sympathetic nerve fibers by a transporter for reuse.
- Both E and NE are released by the adrenal medulla (4:1 ratio) by exocytosis from the secretory chromaffin granules of chromaffin cells. Like other typical endocrine hormones, these hormones are secreted into the circulatory system. This is the source of circulating E.
- Release of NE from sympathetic nerves along visceral blood vessels is the principal source of circulating NE.
E and NE bind with different affinities to adrenergic receptors, which are GPCRs coupled to different signaling G proteins and stimulating different intracellular pathways. The table below shows that each of the major families of heterotrimeric G proteins is activated by one of the adrenergic receptors. This material will be essential in preparation for the ANS Pharmacology sessions that will follow soon. More complete tables are at the end of the chapter.
Table 2: Location and coupling of adrenergic receptors.
Name of Receptor | One typical location | Couples to G-protein α-subunit type |
α1 | smooth muscle | Gαq (smooth muscle contraction) |
α2 | on presynaptic side of sympathetic nerve varicosities | Gαi (inhibits excessive NE release from nerve varicosities, a function called autoinhibition) |
β1 | heart, juxtaglomerular cells | Gαs (promotes contractility |
β2 | smooth muscle | Gαs (smooth muscle relaxation) |
β3 | fat cells bladder cells |
Gαs (lipid breakdown) Gαs (bladder relaxation) |
Learning Objective #3: Compare nicotinic and muscarinic acetylcholine receptor activation and identify acetylcholine receptor types and their effects on various target organs.
Distinguishing nicotinic and muscarinic ACh receptors
There are two major categories of AChRs: nicotinic (nAChR) and muscarinic (mAChR). Except that they both live in the plasma membrane and bind ACh, nicotinic and muscarinic receptors are quite different in structure, function, and pharmacology. Both have many isoforms (genes).
Nicotinic nAChRs are fast, ligand-gated cation channels opened directly by binding of ACh. Opening these channels can depolarize a cell to 0 mV. (Technically, being permeable to Na+ and K+, their reversal potential is around 0 mV.) Outside the central nervous system, nACHRs are located both at the skeletal neuromuscular junction (NMJ) and at synapses onto postganglionic neurons in all ANS ganglia and onto adrenal chromaffin cells. The nAChR isoforms (genes) expressed in nerve versus muscle are different, so it is possible to use drugs to block the neuronal and muscle receptors selectively. However, many nAChR pharmacological agents will affect both, including ACh, which is the natural agonist for both. The ACh binding sites of nAChRs are blocked by the paralytic drug curare and related compounds. The nicotinic name comes from the early discovery that nicotine and smoke from tobacco can activate these receptors as well. There are no nAChRs on cardiac muscle, smooth muscle, or on non-nerve/muscle tissue cells such as liver, kidney, epithelia, etc.
Muscarinic mAChRs are G-protein coupled receptors (GPCRs), not ion channels. They are located on the target tissues of parasympathetic innervation and at the sympathetic sweat gland—indeed on most cells of the body. There are five types, but the two primary receptor types to consider here are M2 and M3. All are inhibited by the drug atropine (an antagonist competing with ACh at the binding site) from deadly nightshade. Atropine does not affect nicotinic receptors.
M2 couples to Gαi. It slows heart rate through both:
1) activation of a K+ channel via Gβγ subunits, leading to hyperpolarization of pacemaker, and
2) turning off the formation of the stimulatory cAMP second messenger through inhibiting adenylyl cyclase via Gαi.
M3 couples to Gαq. The following three actions all result from the ability to elevate intracellular Ca2+ through Gq and the PLC/IP3 pathway.
• Contracts smooth muscle.
• Stimulates glands to secrete (including eccrine sweat glands).
• Elevates nitric oxide (NO) production in endothelial cells leading to smooth muscle relaxation (vasodilation).
Synthesis and cycling of ANS neurotransmitters
We now review synthesis and degradation of the neurotransmitters E/NE and ACh (see also ANS Pharmacology sessions):
NE and E are catecholamines, members of the biogenic amine family. They are released from postganglionic varicosities, taken up again by a Na+/Cl−-coupled NE uptake transporter, and degraded by cytoplasmic monoamine oxidase (MOA).
You do NOT need to memorize their chemical structures.
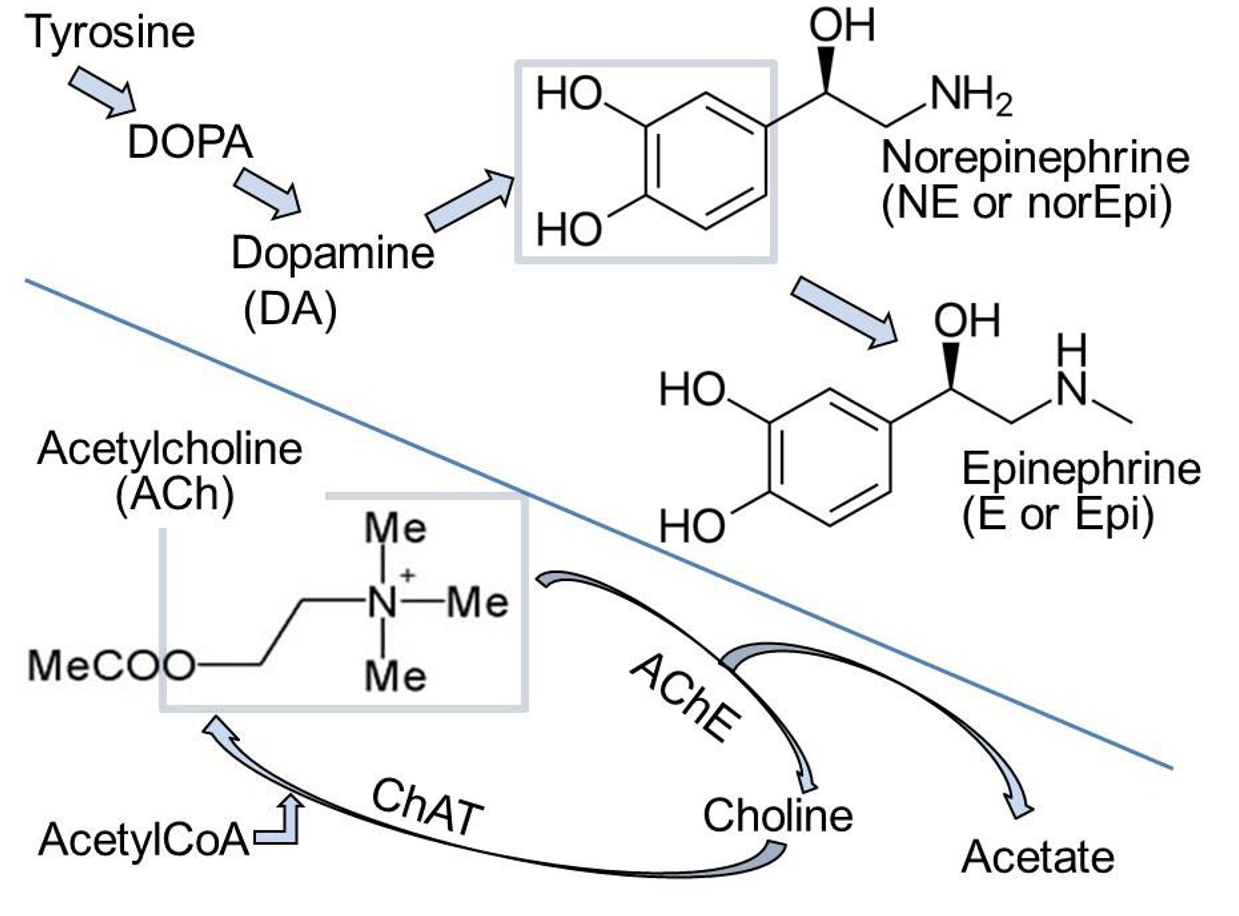
• Synthesis (Figure 4): The precursor tyrosine is taken up into varicosities by a Na+/tyrosine cotransporter. Cytosolic enzymes convert tyrosine to DOPA and then to dopamine.
• Dopamine is transported into secretory vesicles.
• Inside vesicles, dopamine is converted to NE.
• In the adrenal gland, NE leaks out again to the cytosol where NE is methylated to form E (Figure 4), and E is re-transported into the giant secretory vesicles called “chromaffin granules” in the adrenal gland.
NE is normally released from vesicles by exocytosis, but NE also leaks from nerve into serum. This leak is increased if cytoplasmic monoamine oxidases (MAOs) are inhibited by MAO inhibitors (MAOIs).
ACh is an ester formed from choline and acetate (Figure 4). Choline and Acetyl-CoA are combined by choline acetyl transferase (ChAT) in the presynaptic terminal. ACh is then transported into secretory vesicles and released at varicosities and synaptic terminals. ACh is inactivated in the extracellular space by hydrolysis back to choline and acetate by the highly active extracellular enzyme acetylcholinesterase (AChE) present in all cholinergic tissues. The enzyme AChE is the target of nerve gases, such as CX, and organophosphate insecticides. Death is by accumulation of excess circulating ACh and, for humans, by exaggerated parasympathetic action. Choline is recycled by uptake into the nerve by a Na+-coupled cotransporter–conservation. There, choline acetyl transferase resynthesizes ACh. Of all the steps mentioned here, the AChE enzyme will be the most important one for you to remember now.
You do NOT need to remember the structures.
Learning Objective #4: Identify the role of ATP and nitric oxide in smooth-muscle relaxation and blood-vessel dilation.
In addition to NE, E, and ACh, the neurotransmitters ATP and NO (nitric oxide) can play important roles in the ANS.
NO, nitric oxide, is a hydrophobic gas (like CO, carbon monoxide) that diffuses readily from one cell to the next. It relaxes vascular smooth muscle. NO is synthesized inside cells by nitric-oxide synthase (NOS) in response to Ca2+/calmodulin following cytosolic Ca2+ rises. The diffusible NO gas stimulates guanylyl cyclase inside cells. This enzyme related to adenylyl cyclase generates cyclic-GMP (cGMP), which activates protein kinases that promote smooth muscle relaxation increasing blood flow. Figure 5 illustrates two possible sources of NO. In the male and female genitalia, NOS seems to be in the varicosities of the postganglionic nerve fibers, and so NO synthesis is increased by the same presynaptic Ca2+ rise that also mediates exocytosis of vesicles of ACh. The NO potentiates blood flow during sexual arousal. Viagra and other erectile dysfunction drugs act by prolonging the lifetime of cGMP (stopping its breakdown by a PDE) and hence promoting vascular smooth muscle relaxation. In the cardiac coronary circulation, NOS is in the endothelial cells that line the blood vessels. Calcium rises through ACh acting on endothelial M3 receptors. NO then diffuses to the blood vessel smooth muscle to allow vasodilation and enhance coronary blood flow. Some nitro drugs that release NO, such as nitroglycerine, are used clinically to improve coronary circulation.
ATP is obligatorily co-packaged and co-released with NE, E, and ACh as the polyvalent anion that counters the positive charge of these neurotransmitters inside the synaptic vesicles and chromaffin granules. It is co-released during exocytosis as a cotransmitter. The released extracellular ATP can activate purinergic receptors that are ligand-gated non-selective cation channels. Activation of such purinergic receptors initiates contraction of smooth muscle in arterioles and in the vas deferens.
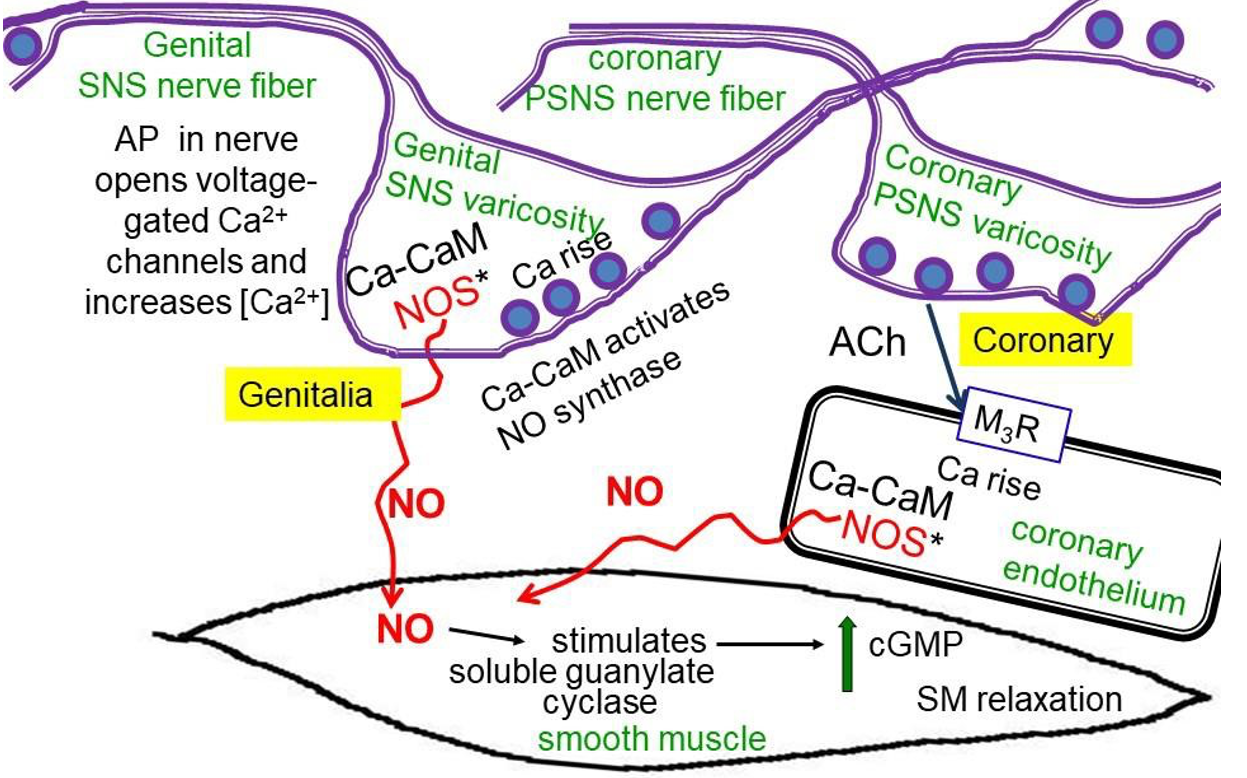
Learning Objective #5: Describe the Baroreceptor reflex in response to high or low blood pressure.
An autonomic reflex regulating blood pressure
The peripheral somatic system has reflexes such as the familiar tendon-jerk reflex involving a short and involuntary arc through the spinal cord leading to motor output. Similarly the ANS has short and involuntary reflex arcs from peripheral receptors through the CNS and out again through ANS ganglia to ANS effectors (Fig. 4). We now describe the Baroreceptor Reflex, an autonomic reflex that regulates blood pressure. Like all reflexes it has three parts:
- (i) afferent input from sensory receptors,
- (ii) a processing center in the spinal cord or brain, and
- (iii) efferent output, which in the case of the baroreceptor reflex is mediated by efferent activity in sympathetic and parasympathetic nerve fibers and adjusts blood pressure.
Here’s how the baroreceptor reflex works:
- Sensory receptor afferent input. Baroreceptors are stretch-sensitive mechanoreceptors in the walls of the aortic arch and carotid sinus that monitor blood pressure by responding to changes in the tension of the arterial wall. Stretch activates them.
- Central processing center. Afferent input carried by spike activity (action potentials) in baroreceptor axons increases with elevated blood pressure and is sent to the medulla of the brainstem for integration.
- Efferent outflow. Increased input from receptor afferents excites the parasympathetic NS and inhibits the sympathetic NS, which lowers blood pressure.
The baroreceptor reflex stabilizes blood pressure by adjusting the activity of the sympathetic NS and the parasympathetic NS. For example, a drop in blood pressure reduces tension in the walls of the aortic arch and carotid sinus, decreasing excitation of the stretch sensitive baroreceptors that results in a corrective increase in segmental sympathetic outflow and a decrease in parasympathetic outflow (in the Vagus nerve) as illustrated in Figure 6.
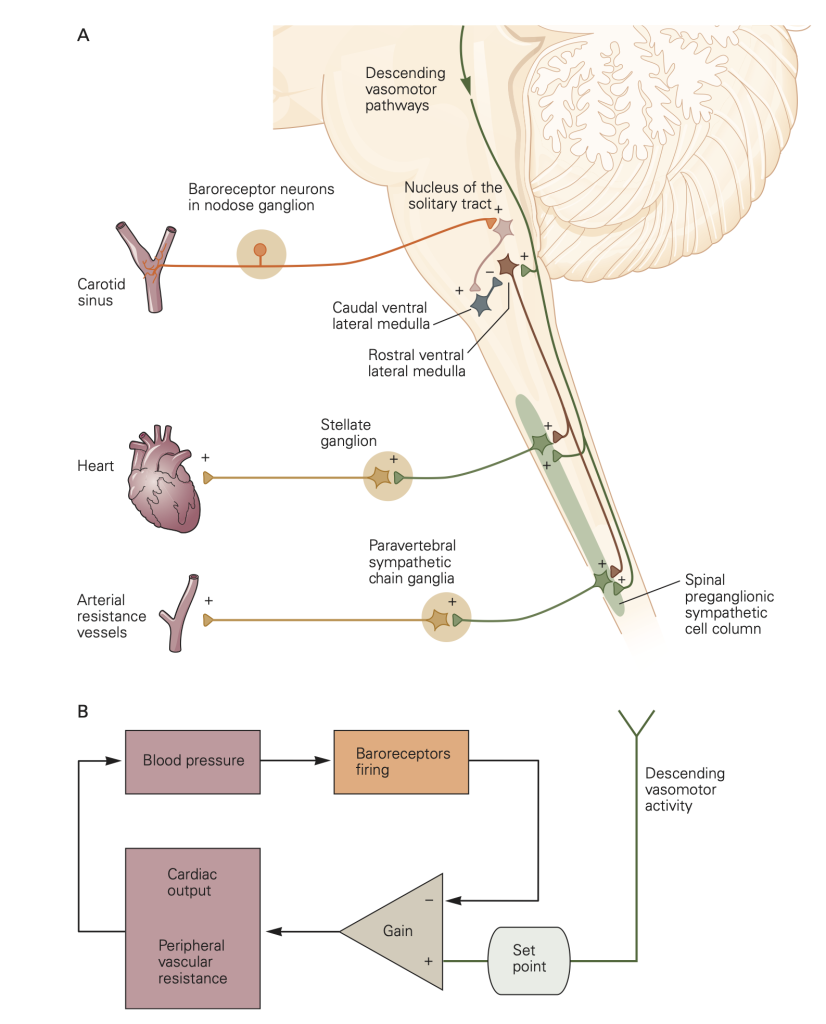
Learning Objective #6: Define orthostatic hypotension and discuss how the baroreflex counters it.
Orthostatic hypotension (postural hypotension) is a sudden decrease in blood pressure that occurs when a person quickly assumes a standing position and blood pools down in the lower extremity under the influence of gravity.
The gravitational redistribution of blood volume reduces blood flow to the heart causing the amount of blood pumped by the heart to decrease, a drop in arterial blood pressure, less flow to the brain, and potential dizziness. The baroreceptor firing rate falls and triggers the sequence of events in the baroreceptor reflex . The increased sympathetic outflow and reduced parasympathetic outflow speed the heart rate and constrict the vessels. This “autonomic reflex” boosts the blood pressure and baroreceptor spike activity in a manner that ultimately restores blood pressure to its normal resting set point. It is involuntary and quick. You are not aware that it is happening except that you may realize that it is good advice to the elderly to support themselves for a few seconds after getting out of bed or after standing up from a crouching position–like after retrieving objects off a bottom shelf.
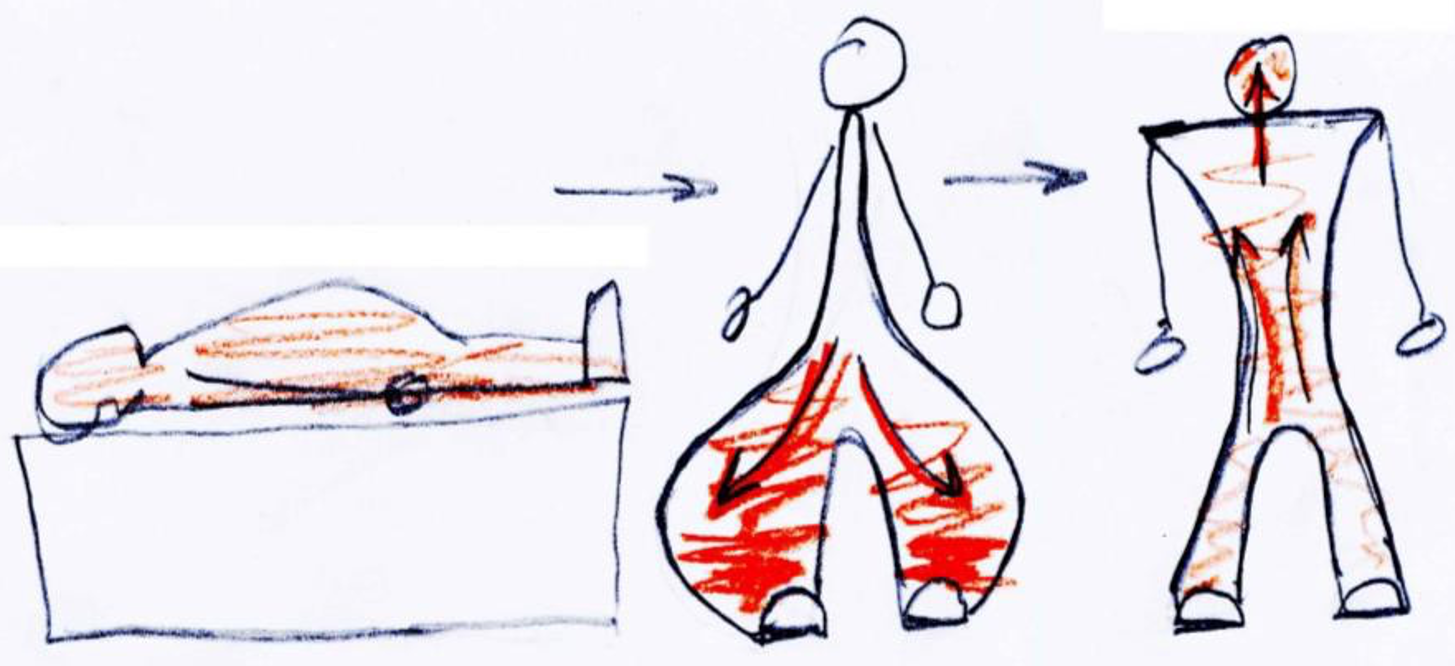
Conclusion
The autonomic nervous system controls many involuntary actions including regulatory, homeostasis, and housekeeping functions of the body. The sympathetic and parasympathetic branches using NE and ACh, respectively, usually exert opposite physiological effects: flight or fight versus rest and digestion. They can be understood from the different G proteins that are engaged. Many patients arriving in the emergency room need immediate pharmacological intervention of their autonomic function.
Table 3: Clinically relevant ANS receptors and their signal-transduction mechanisms (begin to memorize them).
|
G-protein |
2nd messenger |
Effect |
Location |
Adrenergic: |
|
|
|
|
Alpha1* |
Gq |
PKC, Ca2+ |
Contr./secrete |
Smooth muscle/ glands |
Alpha2* |
Gi |
Gβγ- sub- units and -cAMP |
varies |
On presynaptic side of adrenergic nerve terminals, acts to inhibit subsequent NE release from the terminal–called autoinhibition. |
Beta1 |
Gs |
+cAMP |
Increase heart rate & contractility |
Heart muscle, kidney juxtaglomerular cells (renin release), fat cells |
Beta2 |
Gs |
+cAMP |
Relax |
Smooth muscle, glands & enteric neurons |
Beta3 |
Gs |
+cAMP |
Relax; prevent urination |
Bladder; also fat cells (lipolysis and thermogenesis) |
Muscarinic: |
|
|
|
|
M2 |
Gi |
-cAMP |
Slow heart rate |
Primarily heart; a few in smooth muscle that cause contraction |
M3 |
Gq |
PKC, Ca2+ |
Contract or relax** |
Smooth muscle, salivary glands, eyes, vascular endothelium |
*There are 3 distinct alpha1 receptor isoforms (genes) and 3 distinct alpha2 isoforms; the distinctions are beginning to be exploited clinically and you will learn more in later blocks.
** Because the M3 receptor is Gq-coupled and mediates an increase in intracellular calcium, it typically causes contraction of smooth muscle, such as bronchoconstriction. However, in the vasculature, activation of M3 receptors on vascular endothelial cells accelerates synthesis of NO, via Ca2+ which diffuses to adjacent vascular smooth muscle cells and relaxes them (Figure 5), explaining the contrasting parasympathetic effects on vascular tone versus bronchiolar tone. Direct stimulation of vascular smooth muscle M3 receptors mediates vasoconstriction in pathologies that disrupt the vascular endothelium. M3 receptors stimulate secretion in many glands, including the salivary glands and lacrimal glands.
Table 4: Effector organs and ANS innervation. (Use this table to interpret actions of different pharmaceutical agents.)
Effector organ |
Parasympathetic action |
Target muscarinic receptor |
Sympathetic action |
Target adrenergic receptors |
Eye |
|
|
|
|
1) Lacrimal glands |
Secretion |
M2/M3 |
– |
– |
2) Pupil sphincter (para) or dilator/radial (symp) |
Constriction |
M3 |
Dilation |
Alpha1 |
Ciliary muscle (para) Ciliary process / Aqueous humor |
Contraction – |
M3 – |
– Increase flow |
Beta2 |
Heart rate and output |
Decrease in rate |
M2, M4 |
Increase in rate & contractility |
Beta1 |
Arterioles GI tract, genitals Almost all others In Skeletal muscle |
Dilate/ relax – – |
*M3 / via nitric oxide (NO) |
Constrict Constrict Relax |
Alpha1 Alpha1 Beta2 |
Lungs Bronchial muscle |
Constrict |
M3 |
Dilate |
Beta2 |
GI Tract Mobility and secretion Sphincters |
Increase/excite Relax |
M3, M1 M3 |
Decrease/inhibit Constrict |
Beta2, alpha2 Alpha1 |
Sweat glands |
– |
|
Secretion |
**mAChR (M3) or alpha1 / beta2 |
Adrenal Medulla |
– |
|
Secretion |
**nAChR |
Bladder Bladder wall |
Contract |
M3 |
Relax |
Beta2 |
Reproductive organs |
Erection, vaginal lubrication |
*M3 / via NO |
Ejaculation |
Alpha |
Exocrine glands |
Salivation |
M1, M3, M4 |
|
|
*Nitric oxide’s (NO) role in arteriole dilation is discussed in footnote to Table 3 and in Figure 5.
**Note two non-adrenergic exceptions for sympathetic receptors: nAChR is the type of receptor found on all postganglionic neurons of the ANS (different isotype from nAChRs found on skeletal muscle). Sweat glands have either mAChRs or adrenergic receptors, not both.