15 Skeletal Muscle Histology
Skeletal Muscle Fibers
Skeletal muscle cells are unique, very large and long cells that are usually referred to as skeletal muscle fibers. As shown in the schematic figure comparing skeletal muscle, cardiac muscle, and smooth muscle, the diameter of a skeletal muscle fiber is comparable to the length of a cardiac or smooth muscle cell (about 100 μm) while the length of a skeletal muscle fiber can be many centimeters.
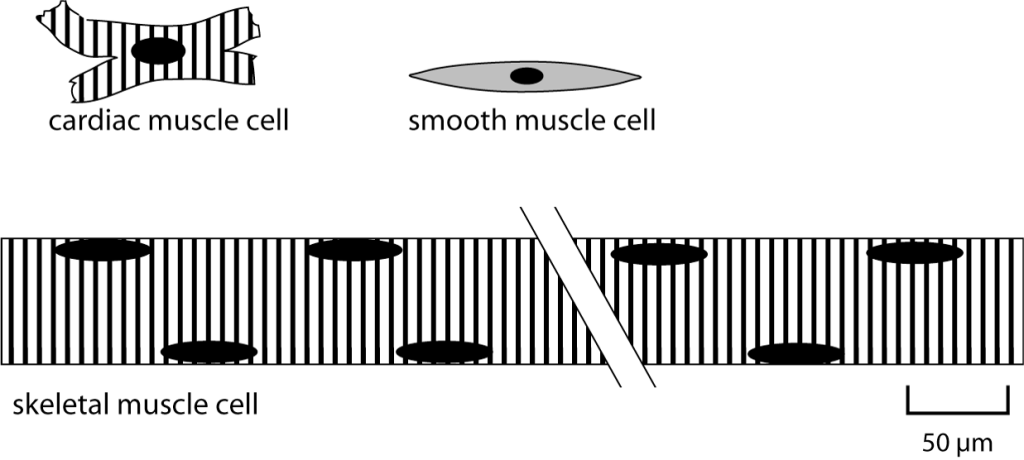
Skeletal muscle fibers are multinucleate. The nuclei are located at the edge of the cell, as depicted above. Skeletal muscle fibers develop from the fusion of embryonic precursor cells called myoblasts. In adult muscle, there continue to be satellite cells, which are stem cells that can be activated to divide and become myoblasts if needed for skeletal muscle growth or repair.
Another unique feature of skeletal muscle fibers is that they are striated. The stripes, or striations arise because of the alignment of myofibrils within the skeletal muscle fiber. The skeletal muscle cell, itself a tube, is packed full of tubes–the myofibrils. Myofibrils are the organelles that contain the contractile proteins actin and myosin. When skeletal muscle hypertrophies (grows) in response to training, it increases in diameter by adding myofibrils. Adding myofibrils increases strength because more contractile proteins working increases the force that the muscle fiber can produce.
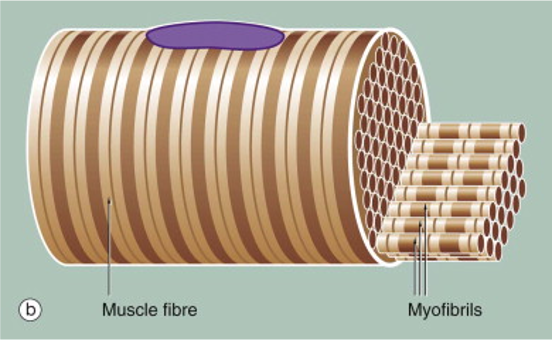
At high magnification in the light microscope, skeletal muscle fibers cut in cross-section appear polygonal. Each of the dots within the cell corresponds to an individual myofibril.
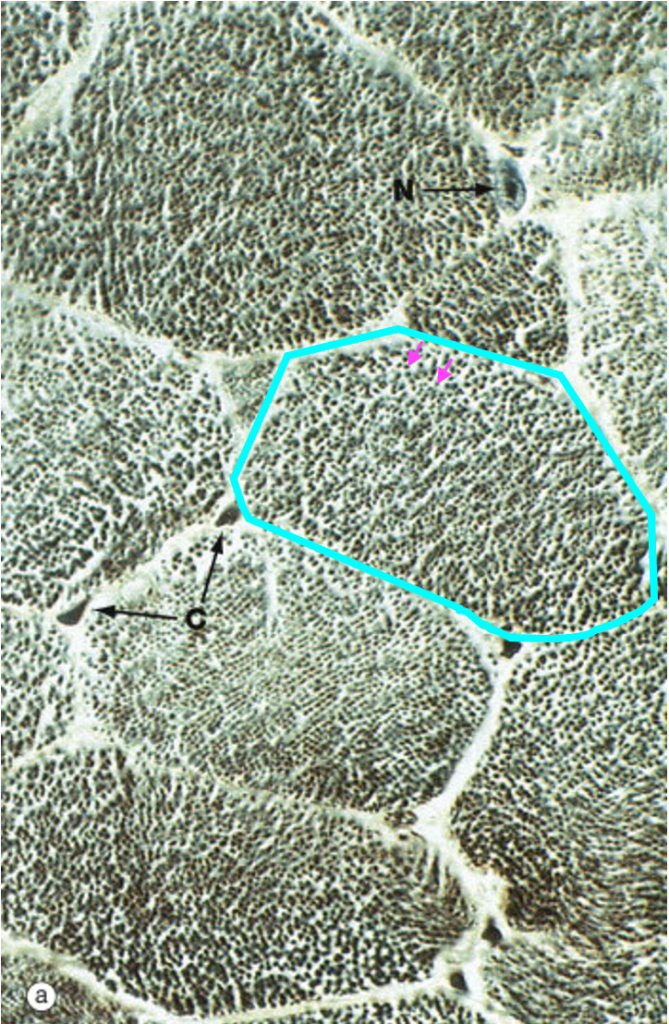
Each myofibril is made up of many sarcomeres, linked end to end to end. The figure below provides a cutaway view of myofibrils and other structures within a skeletal muscle fiber. This figure illustrates how the sarcomere, the contractile unit of the myofibril, relates to the striation pattern that can be seen when viewing skeletal muscle in a longitudinal section.
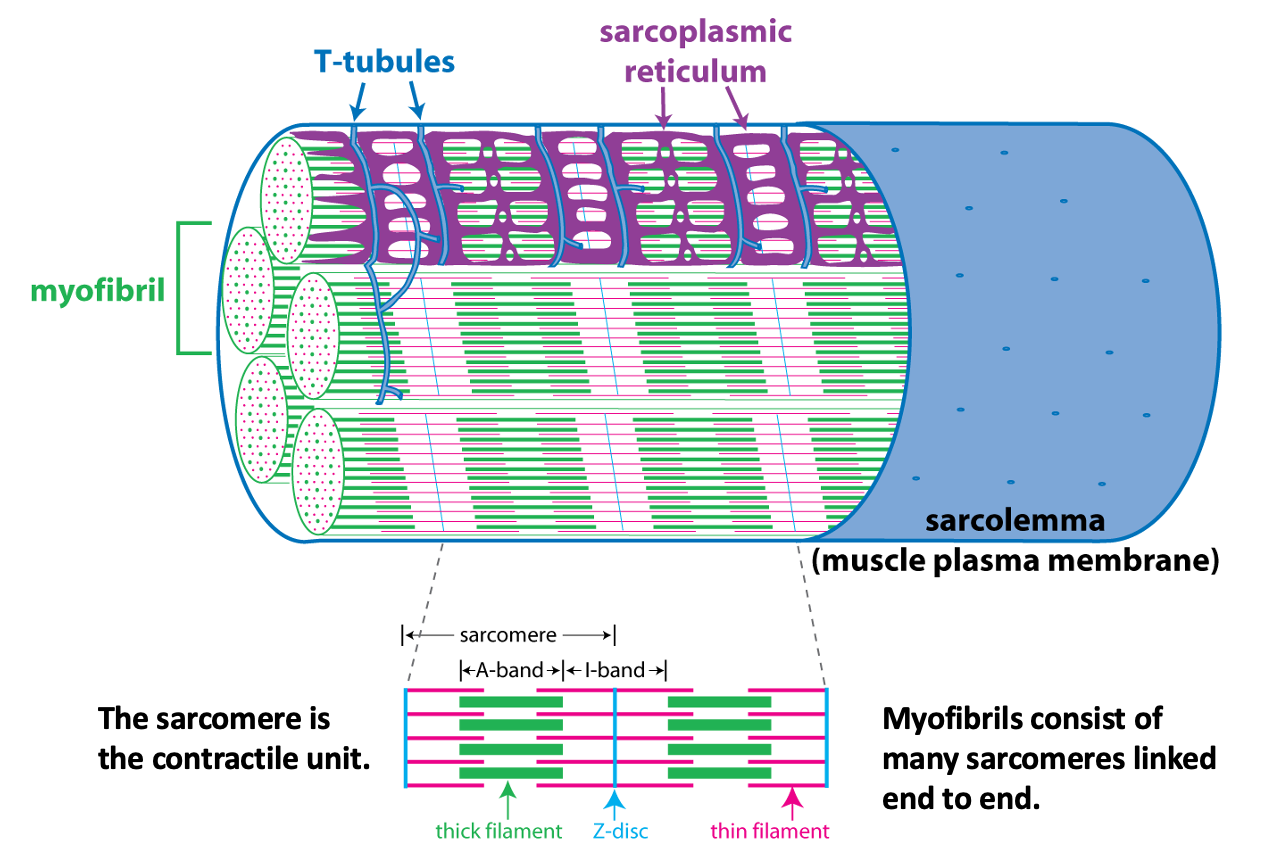
The next figure shows a light micrograph and two electron micrographs (EMs) of skeletal muscle fibers to illustrate the details of the alternating dark and light stripes of skeletal muscle striations. The dark stripes are called A bands, while the light regions are called I bands. At the center of the I band is a thin dark line called the Z disc. (Optional: “A” and “I” stand for anisotropic and isotropic, terms that refers to how these regions scatter light when viewing the muscle fiber with polarized light microscopy.)
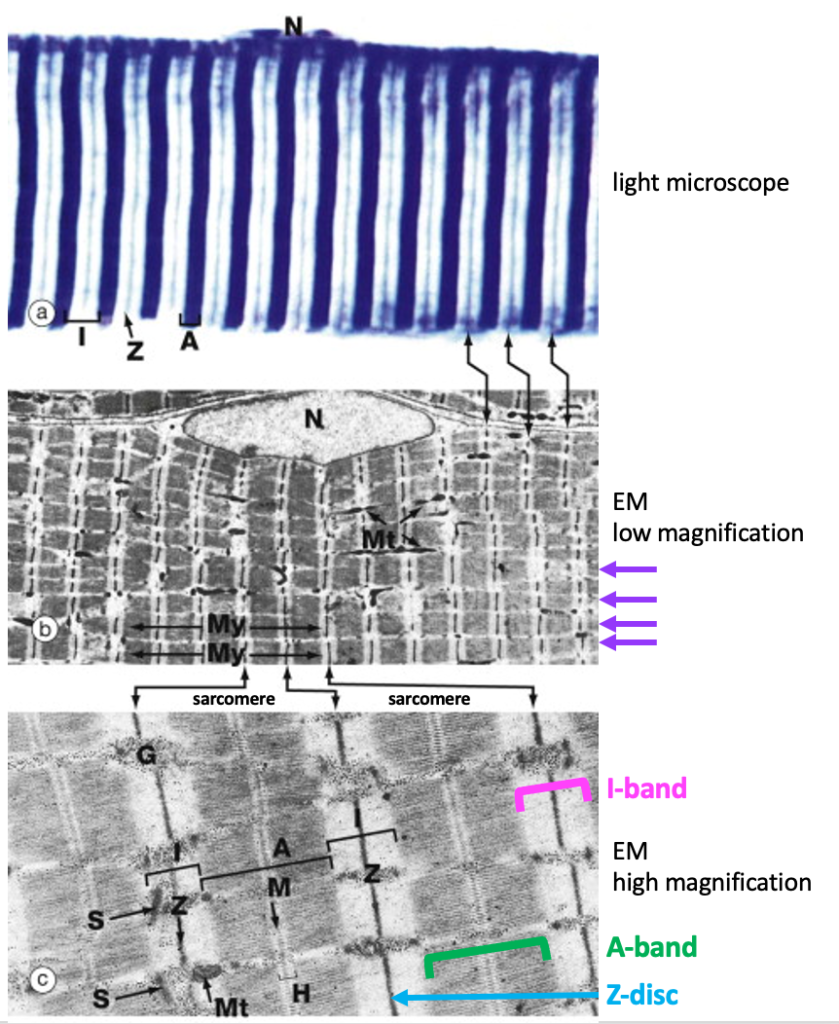
The next figure relates the A band and the I band to the structure of the sarcomere. The sarcomere consists of a set of thin filaments of actin (pink) arrayed around thick filaments of myosin (green). The filaments are anchored at the z-disc(blue).
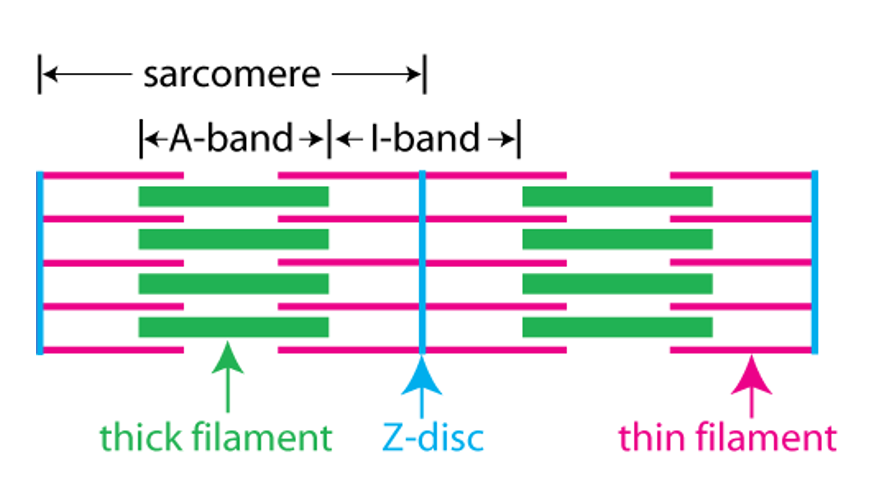
The A band corresponds to the region where there are thick filaments. The I band corresponds to the region where there are no thick filaments.
Below is a light micrograph of a section through the tongue. The tongue is a good muscle to look at because it contains multiple bundles of skeletal muscle fibers in a complex arrangement. The figure below shows some fibers cut in cross-section (blue arrows) and some cut in longitudinal section showing striations (yellow arrows). Because this is a lower magnification, t is only possible to see A bands and I bands and not Z-discs.
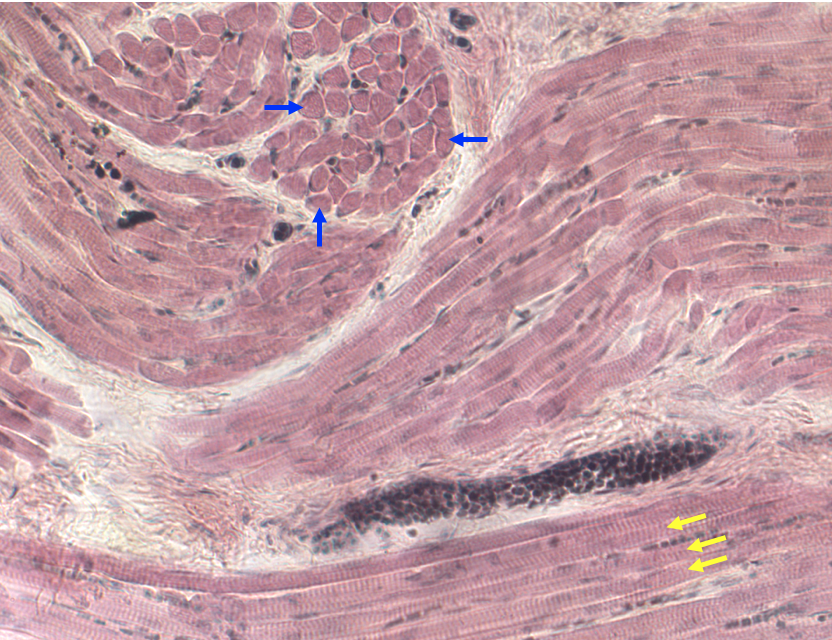
Skeletal Muscle Contraction
Movement and force generation by skeletal muscle is achieved by muscle fibers pulling on connective tissue; usually tendons that are attached to bone. Muscle fibers pull on their ends by contracting (shortening). Two groups of scientists working in the 1950’s determined that skeletal muscle contraction involved the movement of the thin filaments relative to the thick filaments. This is called the sliding filament mechanism of contraction.
The figure below shows the key experiment performed by Hugh Huxley and Jean Hanson.
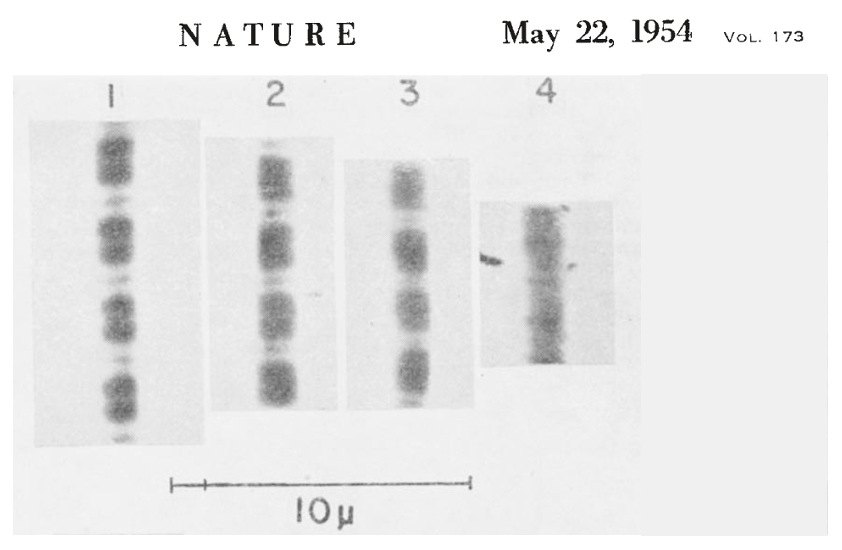
Number 1 shows the myofibril at its resting length, and numbers 2, 3, and 4 show it at later times following the addition of ATP. ATP is what fuels crossbridge cycling, the biomechanical process that underlies muscle contraction (see below). What they observed is that the width of the I band decreased as the myofibril contracted, but the width of the A band stayed the same. Knowing the structure of the sarcomere, and that the A band contains the thick filaments, they hypothesized that what occurred during contraction was that the thin filaments on each side of the sarcomere were pulled toward the center of the sarcomere. This is illustrated in the figure below (sarcomeres oriented horizontally, unlike the above figure where they are oriented vertically).
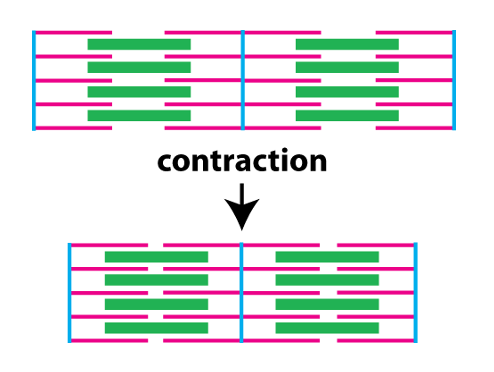
The biomechanical process underlying muscle contraction is called crossbridge cycling. The next figure illustrates sarcomeres, but provides more detail about the structure of the thick and thin filaments.
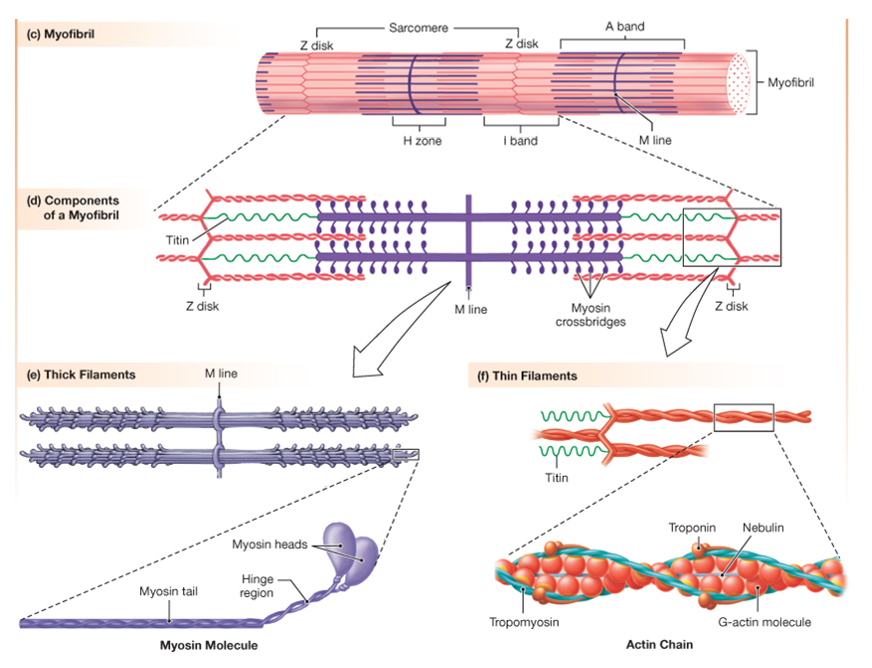
The thin filaments consist of two chains of filamentous actin wound around each other. Each actin monomer (G-actin in the figure) has a binding site for myosin. Protruding from the thick filament are the myosin heads. Each myosin head can bind to actin in the thin filament, and is also an ATPase, meaning it can hydrolyze ATP to cause conformational changes in its protein structure. During contraction, the myosin heads undergo repeated cycles of ATP hydrolysis, conformational changes, and binding to actin (crossbridge cycling) that slide the thin filaments toward the center of the sarcomere.
The schematic cutaway drawing of a skeletal muscle fiber illustrates three other important structures involved in muscle contraction.
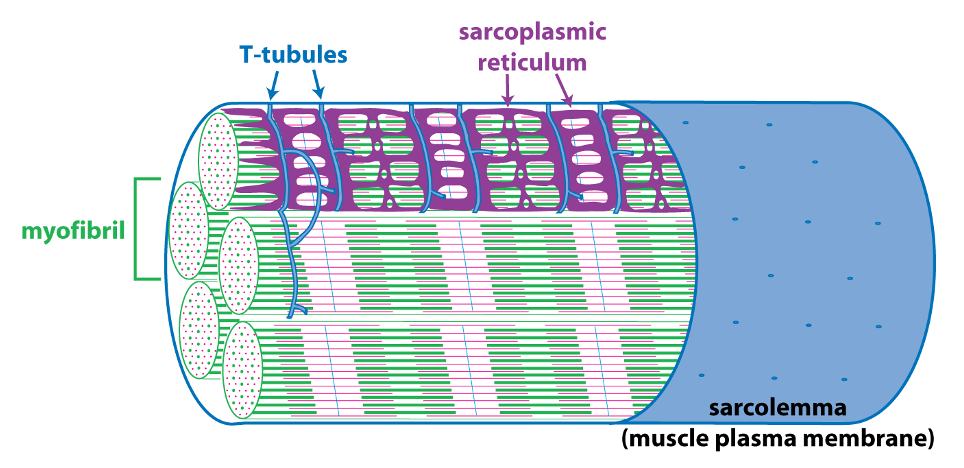
The signal for a muscle to contract comes from the somatic motor neuron (see below) that excites the skeletal muscle fiber through release of the neurotransmitter acetylcholine at the neuromuscular junction. Acetylcholine binding to its receptors causes a large end plate potential that triggers an action potential. The skeletal muscle action potential conducts along the surface of the sarcolemma (muscle fiber membrane) and into the interior of the skeletal muscle fiber via membrane invaginations called T-tubules. The T-tubules form a close association with the sarcoplasmic reticulum, the membrane-bound organelle that stores and releases Ca++. Excitation-contraction coupling, is the linking of the muscle action potential (excitation) to Ca++ release from the sarcoplasmic reticulum because Ca++ is the regulator of muscle contraction. Excitation contraction coupling involves an interaction between a voltage-sensitive protein in the membrane of the T-tubule that is directly linked to a Ca++-release channel protein in the membrane of the sarcoplasmic reticulum.
Neuromuscular Junction
As we learned previously when studying the organization of the peripheral nervous system, skeletal muscle is innervated by somatic motor neurons. “Innervation” here means that the skeletal muscle cell receives a synaptic input from the somatic motor neuron. This large, specialized synapse is called the neuromuscular junction.
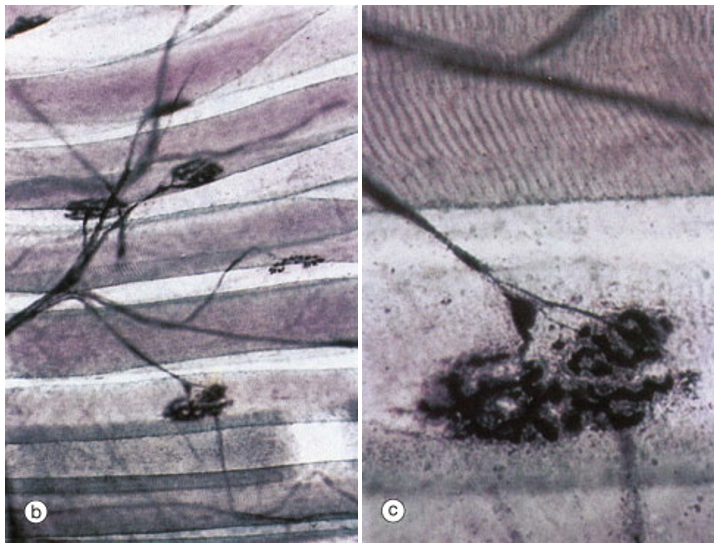
The figure above shows a somatic motor neuron branching to form synapses with several different muscle fibers. The large disc-like shape is characteristic of the neuromuscular junction. In the right-hand panel, which is a higher magnification view of the lowermost synapse, one can clearly see striations, indicating that the large tubular cells are skeletal muscle fibers.
This figure illustrates that while each skeletal muscle fiber is innervated by only one somatic motor neuron at a large neuromuscular junction, each skeletal muscle fiber branches to innervate multiple skeletal muscle fibers. Recall that the neuromuscular junction has a high safety factor. So much neurotransmitter is released by the somatic motor neuron that the endplate potential (postsynaptic potential in the skeletal muscle fiber) is so far above threshold that an an action potential in the somatic motor neuron always leads to an action potential in the skeletal muscle fiber. Thus all the muscle fibers innervated by one somatic motor neuron will all be activated when that neuron is activated. The motor unit is the somatic motor neuron and all the skeletal muscle fibers that it innervates.