CHB Chapter Uploads
4 Test Import 5/5/25 Introduction to Endocrine and Hormone Signaling
Type your textbox content here.
Key Takeaways
Type your key takeaways here.
- First
- Second
Learning Objectives
- Describe the characteristics that distinguish endocrine organs from non-endocrine organs
- Define hormones and describe the signaling pathways through which hormones act
- Describe the characteristics of peptide, steroid, and thyroid hormones
- Describe the different means through which hormone action can be regulated
- Explain the concept of feedback regulation and disorders of feedback regulation
- Interpret lab results to identify disorders of simple feedback regulation
- Interpret lab results to differentiate between primary, secondary, tertiary dysfunction of pituitary feedback regulation
Type your textbox content here.
Learning Objectives
Introduction
The “endocrine system” is a collection of chemical mediators, termed “hormones,” that conveys information from one point to another. Endocrine organs secrete hormones into the circulation, where they travel through the blood to target organs some distance away. This is distinct from “paracrine” actions, where hormones diffuse from their site of production to act on neighboring cells or “autocrine” action, with some hormones acting on their own secreting cells. “Neuroendocrine” action involves hormone release triggered by nerve synapses that secrete their neurotransmitters into the blood, to travel to distant endocrine cells (figure 1).
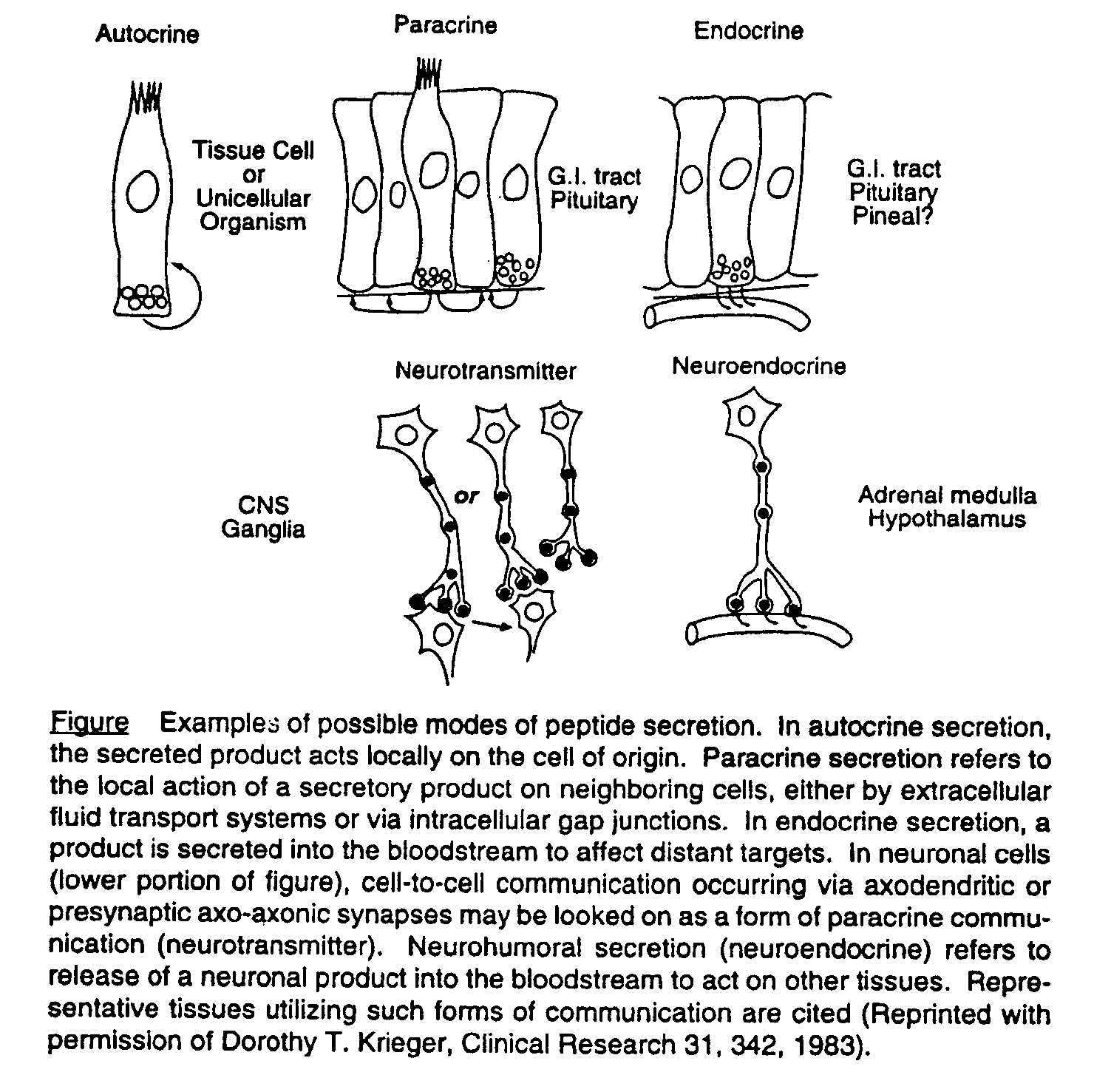
Endocrinology is concerned with the study of hormones. Integral to the study of endocrinology is an understanding of the regulation of hormones. The synthesis and release of these hormones is controlled by different factors, including the hormone itself, or by an effector molecule (e.g. serum glucose controls insulin secretion, which in turn controls glucose levels). This regulation helps to maintain hormone levels in a normal range. Endocrine disorders result when this regulation is disrupted, either by dysfunction at the level of the target organ, or abnormal levels of the hormone acting at the target organ.
Hormones regulate reproduction, growth and development, and maintenance of the internal environment. They control energy production, utilization and storage. They have a key role in the normal function of nearly every system in the body. Hormones are fundamental for maintaining homeostasis of body fluids, blood pressure and body temperature.
The goal of this chapter is to introduce you to the “endocrine system” and the concepts of how hormones regulate physiological function. In subsequent chapters, we will discuss the regulation of each endocrine system in detail, as well as the disorders that result when that regulation goes awry.
Hormone synthesis and actions
Types of hormones
Hormones fall into two broad categories: peptide hormones and steroid hormones. Thyroid hormones are unique and in a class of their own and are similar to steroid hormones in many ways. The majority of hormones are peptide hormones or amino acid derivatives. The remainder are steroid hormones or steroid derivatives.
Peptide hormones are composed of polypeptides chains of amino acids. Synthesis involves several steps occurring in the nucleus and the cytoplasm (endoplasmic reticulum and golgi apparatus). The peptide hormones are synthesized as preprohormones in the endoplasmic reticulum where they are also converted to prohormones. The golgi body is responsible for cleaving other peptide sequences, thus producing the hormone which is stored in secretory vesicles until needed. Peptide hormones are hydrophilic, circulate freely in the plasma, and bind to cell surface receptors. They generally have fast effect through change in protein activity (but can also affect gene transcription). Some examples of peptide hormones are insulin, glucagon, all of the anterior pituitary hormones, PTH, catecholamines, and the posterior pituitary hormones.
Steroid hormones are synthesized from cholesterol. They are not stored but are released immediately after synthesis. In contrast to peptide hormones, they are hydrophobic and are bound to transport proteins in the plasma and can cross the cell membrane, where they bind to an intracellular receptor. Availability of transport proteins will affect the total measured level of the hormone (but not the free, or biologically active, level). Transport proteins can be affected by several factors: malnutrition, liver disease, and kidney disease lead to decreased transport proteins. Estrogen often leads to increased transport proteins. The effects of steroid hormones lead to alterations in gene expression, meaning action is slower in onset. Examples of steroid hormones are cortisol, aldosterone, estrogen, progesterone, testosterone, and vitamin D.
Thyroid hormones have characteristics of both steroid and peptide hormones. They are amino acid (tyrosine) derivatives that are stored prior to release. They are protein bound for travel through the circulation and act on intracellular (nuclear) receptors.
There are several characteristics shared by hormones, that help control the specificity of their action. Hormones are present in the circulation in low concentration. The plasma concentration of steroid and thyroid hormones exist in pM and µM amounts, while that for peptide hormones is generally between 1 and 100 fM (10-15M). They are directed to sites of action by specific mechanisms, typically via specific receptors in target tissues that recognize and bind the hormone with high affinity, which limits their actions to specific tissues. Transportation of the hormone via restricted circulation or binding protein can also control plasma levels and direct hormone action. Finally, the synthesis and breakdown of hormones, which can be stimulated or inhibited depending on tissue needs, can also control their action.
Hormone receptors
Hormones have no biologic action without receptors. There are two general classes of receptors that interact with the different types of hormones. The first class of receptors includes cell surface proteins that interact with peptide hormones. These receptor proteins in the plasma membrane of the cell transduce signals that usually involve second messenger molecules within the cell, regulate protein phosphorylation, and/or intracellular calcium fluxes (figure 2A). These signals determine hormone action. As a result of hormone-receptor interaction, the cell will internalize and degrade the bound hormone, thereby regulating its concentration in plasma. The level of hormone in the plasma can also upregulate or downregulate the number of receptors on the cell surface, which is another means of controlling hormone action.
The cell surface receptors of relevance are G-protein couple receptor (GPCR) (Gs subunit and Gq subunit), receptor tyrosine kinase (RTK), and the JAK-STAT pathway. GPCR represent the majority of hormone cell-surface receptors and are 7-transmembrane domain receptors coupled with G-proteins. There are two principal signal transduction pathways involving the G-protein coupled receptors – the cAMP signal pathway and the phosphatidylinositol (PIP) pathway. Table 1 details the cell surface receptors and associated hormones relevant to endocrinology.
Receptor |
Signal Pathway |
Relevant Hormones |
GPCR-Gs |
cAMP |
FSH, LH, ACTH, TSH, hCG, PTH, calcitonin, glucagon, ADH (kidneys) |
GPCR-Gq |
PIP |
GnRH, GHRH, oxytocin, ADH (pituitary), TRH, glucagon |
Receptor tyrosine kinase (RTK) |
Downstream phosphorylation events |
Insulin, IGF-1 |
JAK-STAT |
JAK and STAT |
GH and prolactin |
Table 1. Summary of hormone cell surface receptors
The second class of receptors includes cytoplasmic proteins that interact with steroid hormones and thyroid hormone, as we will discuss in a later chapter. These are the water insoluble hormones (hydrophobic) that require binding proteins for transport. Their lipid solubility enables these hormones to pass through the plasma membrane into the cell, where intracellular binding proteins (receptors) carry the hormone to its target site in the nucleus where it interacts with chromatin to influence gene transcription (figure 2B).
Figure 2. A) example of a cell surface receptor (left), in this case a G-protein coupled receptor that acts through cAMP as a 2nd messenger. B) example of an intracellular receptor (right), which bind the hormone in the cytoplasm of the cell and transports it to the nucleus where it affects gene transcription.
Hormone transport
- Hormone transport is another mechanism that can control the action of hormones. One way this is accomplished is by delivering a hormone to specific target tissues through direct delivery within a restricted circulation. This allows for higher levels of hormone to reach the target tissue and maintains low hormone levels systemically. An example of this is the hypophyseal portal system, where hypothalamic hormones are delivered in relatively high concentration to the pituitary gland, while very low levels of these hormones are present in the systemic circulation. This limits action of the hypothalamic proteins to receptors present in the pituitary gland.
Hydrophilic (water-soluble) hormones are transported in plasma and require no specific transport mechanism. The water insoluble (hydrophobic) hormones however require carrier proteins. Only the free or unbound hormone can enter cells, so the transport proteins act as reservoirs of hormone. As unbound hormone enters a cell, it is replaced in the plasma by hormone newly released from the carrier protein, which controls the levels of free hormone available to cells. Changes in binding protein levels can affect total hormone levels. Estrogen will increase binding protein production, while malnutrition can lead to lower levels of binding protein. In normal conditions, the endocrine gland will compensate for changes in binding protein by increasing or decreasing hormone production. If there is underlying disease however, the gland may be unable to compensate leading to abnormal free hormone levels.
Hormone metabolism
- The synthetic mechanisms for producing hormones are not unique. Peptide hormones, for example, are synthesized by the same biochemical pathways as other proteins. However, the enzymes needed for hormone synthesis and processing are often present only in certain tissues, which contributes to the specificity of hormones. The appropriate processing machinery may only be present in specific tissues, limiting levels of active hormone to their sites of action. Pro-opiomelanocortin (POMC), for example, is efficiently converted to the active hormone ACTH in the pituitary, but not other areas of the brain. Steroid hormones and catecholamines are synthesized from small-molecular-weight precursors. In the case of steroid hormones, the parent molecule, cholesterol, is modified by sequential cleavage of carbon-carbon bonds and hydroxylations to form the varied products. The enzymes necessary for these actions are present only in the adrenal glands.
- Endocrine glands control the release of hormone into circulation, providing yet another means of regulating hormone levels. This is achieved both through control of hormone synthesis and hormone release. Generally, only small amounts of hormones are stored in a pre-formed state. These serve a critical function in emergencies and periods of stress but are generally depleted within hours to days. When higher levels of hormone are needed, the gland is stimulated to increase both hormone production and hormone release.
Rhythms in the release of hormones are a common feature of almost all endocrine systems. These rhythms can vary over minutes to hours (the pulsatile secretion of luteinizing hormone and testosterone), daily (the circadian variability in cortisol secretion), weeks (the menstrual cycle), or even longer periods (seasonal variability in thyroxine production). Patterns may be different at different stages of life. Thus, the sleep-associated surges of gonadotropin secretion that herald the onset of puberty differ from the rhythms of gonadotropin release seen in adult life. These differences in hormone levels based on time of day, or even time of life, help regulate hormone actions.
Regulation of hormones
Feedback regulation
- The principal stimuli to increase hormone output are changes in the level of a circulating metabolite under hormonal control (such as insulin and glucose) or increases in the blood level of a trophic hormone (such as thyroid stimulating hormone (TSH) which controls thyroid hormone levels). The output of each system (the metabolite or hormone) then feed-back to control the level of the stimulating hormone, and thus control their own production. This process is known as feedback regulation and is one of the distinguishing features of the endocrine system.
- Because the hormone and its product regulate one another, it is necessary to simultaneously measure both the hormone and the stimulating hormone in order to determine if the system is in balance. For instance, elevated insulin, which normally acts to lower blood glucose levels, may be an appropriate response to high glucose levels or indicate insulin resistance, while elevated insulin with low glucose suggests inappropriate insulin production. Understanding feedback regulation is key to interpretation of endocrine labs and will be covered in detail in the following chapters.
Pituitary hormones
- The hypothalamic-pituitary-end organ/effector system is one of the key regulators of hormone action and will be covered in detail in a dedicated chapter. The pituitary produces several trophic (meaning stimulating growth) hormones, which act at endocrine/effector glands to 1) increase hormone production and 2) stimulate cell growth. The pituitary itself is controlled by hormones released by the hypothalamus. The levels of these hormones are tightly controlled via feedback mechanisms. As the endocrine target organ increases hormone synthesis and secretion, the levels are detected at both the pituitary and the hypothalamus, which leads to decreased production of the trophic and hypothalamic hormones. Similarly, as hormone levels decrease, the drop in hormone levels is detected at the pituitary leading to increased trophic hormones. This helps maintain these hormone levels in a narrow range of normal.
In a typical negative feedback loop, the rate of secretion of a specific hypothalamic peptide, or releasing hormone (XRH) and the related, specific anterior pituitary stimulating hormone (XSH) will receive a negative influence when the blood level of the effector hormone (XH) becomes too high (figure 3). The secretion of both XRH and XSH then drop, so that the effector gland receives fewer stimuli to produce XH, resulting in lower blood levels of XH. The converse is true if the level of the effector hormone XH is too low. There is decreased inhibition at the pituitary and hypothalamus, leading to increased XSH and XRH as the system acts to increase XH levels and restore homeostasis.
Figure 3. Basic endocrine feedback loop, where “XH” indicates a hormone of the anterior pituitary, “XSH” is a pituitary hormone that stimulates the release of XH and HRH is a hypothalamic hormone stimulating release of XSH. XH provides “negative feedback” to suppress XSH release from the pituitary and XRH from the hypothalamus.
Non-pituitary hormones (aka Simple Feedback Regulation)
There are other endocrine glands, such as the parathyroid glands or the pancreas, that are not under control of pituitary hormones. These glands are under control of the circulating metabolite (M) they control. These glands release their hormone (XH; insulin or parathyroid hormone) which stimulates a change in the metabolite (M; glucose or calcium) through various mechanisms. This change in M is detected by the endocrine glands, leading to a compensatory change in the release of XH (figure 4). All hormones are regulated in some way by the factors they control. This is a key feature of the endocrine system and one that we will review in detail for each of the endocrine organs we will discuss in this course.
Figure 4. Control of non-pituitary hormones (XH). These hormones regulate the release of a metabolite (M) which in turn inhibits further release of XH.
Endocrine disorders
Endocrine disorders are most commonly due to problems with hormone regulation or hormone action. These can be caused by hormone deficiency, hormone excess or hormone resistance. There can also be problems with hormone transport, which results in abnormal hormone levels, but these conditions do not typically cause pathology.
Hormone deficiency
Diminished or absent hormone secretion can have several causes. One cause is absence or malformation of an endocrine organ, such as congenital hypoparathyroidism (absence of the parathyroid glands). Alternatively, the endocrine organ may develop but lack some enzyme essential for hormone synthesis, as is seen in some adrenal disorders. More commonly, a normal endocrine gland is destroyed by a secondary process. These can include infection, infarction, autoimmunity, tumor compression, or a variety of forms of physical damage including radiation or surgery. Deficiency of the stimulating hormone, as in the case of pituitary dysfunction, can also lead to hormone deficiency.
Hormone deficiency is diagnosed based on low hormone levels on laboratory evaluation.
In the case of the hypothalamic-pituitary-end organ axis, to determine whether the problem is with the endocrine gland itself (primary hypofunction) or with the stimulating hormone (XSH) (secondary hypofunction), additional laboratory tests are needed (table 2). In the case of the pituitary hormones, you would measure the appropriate trophic hormone/stimulating hormone (XSH). While hypothalamic hormones (XRH) are also affected by endocrine hormone deficiencies, these hormones are not measurable in the systemic circulation, so are not used clinically. This will be discussed in more detail in the Pituitary chapter.
Location of abnormality |
XH levels |
XSH levels |
XRH levels |
Endocrine gland |
Low |
High |
High |
Pituitary |
Low |
Low |
High |
Hypothalamus |
Low |
Low |
Low |
Table 2. Changes in hormone levels with hypofunction of the hypothalamic-pituitary-endocrine axis, based on location of the lesion.
Often the biochemical pattern of secondary hypofunction (a dysfunction originating with the pituitary gland) causes confusion for the clinician. This is because the level of XSH may be within the normal range, (therefore interpreted as normal), when in fact it should be elevated because the level of X Hormone is low. Though XSH is “normal” it is relatively (and abnormally) low given the low level of XH, and thus is inappropriately normal.
Another challenge in diagnosing endocrine hypofunction is the pulsatile secretion of many hormones. Hormones with a long half-life that are stable in the serum throughout the day, such as thyroid hormone, can be measured accurately at any time. Hormones with diurnal rhythmic secretion (testosterone) are best measured in the morning to assess under-secretion. Hormones with highly variable pulsatile secretory patterns (ACTH, growth hormone) throughout the day often require dynamic testing to determine adequacy, as it can be difficult to know whether a random low level is just normal variation, or due to underlying pathology.
Hormone excess
Endocrine dysfunction can also result in hormone overproduction. Certain endocrine tumors can act autonomously to produce excess hormone, as is seen with thyroid, pituitary, adrenal, parathyroid, and pancreatic beta-cell tumors. The mechanism that controls normal hormone secretion can also be set at an abnormally high level, or hormone signaling may be constitutively active due to receptor abnormalities, which can be seen with parathyroid glands. Autoimmune processes can produce autoantibodies that act to stimulate hormone production, a common cause of hyperthyroidism. Damage to the gland itself can sometimes cause release of pre-formed hormone. Sometimes tumors in other parts of the body can produce substances that mimic hormones. Finally, patients may be taking excessive hormone either prescribed or in over-the-counter nutritional supplements.
Determining the cause of excess hormone can be difficult, given the many possible etiologies. Similar to diagnosing hypofunction, understanding the regulation of the different hormones is necessary to identify the cause of endocrine hyperfunction. For instance, a pituitary tumor producing too much ACTH would lead to elevated cortisol levels (secreted from the end-organ, the adrenal gland). However, an adrenal tumor producing too much cortisol would also result in elevated cortisol levels. To distinguish between these causes, one would need to measure ACTH, the hormone that controls cortisol production. In the case of the pituitary tumor, ACTH would be elevated. With the adrenal tumor however, the high levels of cortisol would appropriately suppress the ACTH. Thus, measurement of both the hormone or metabolite of interest and the hormone that regulates in production and release is critical to fully understanding conditions of excess hormone production.
Additional tests are sometimes necessary to make a definitive diagnosis of hyperfunction. If the hormone is secreted in a variable fashion throughout the day, a blood test may not accurately measure levels. In these cases, measurement of the hormone or its metabolite in a 24-hour urine collection will provide information on levels over the course of the day. We can use dynamic testing to identify the cause of endocrine hyperfunction. In this case, we try to suppress the abnormal hormone level. This involves giving a patient a substance known to suppress a certain hormone, and then measuring the hormone a short time later. In cases of abnormal excess hormone production, levels will remain elevated.
Hormone resistance
Occasionally patients will have symptoms suggesting a hormone deficiency, but hormone levels are normal or elevated. This can be due to hormone resistance. Production of an abnormal hormone can prevent the hormone from binding and signaling via its receptor. Hormone precursors may be incompletely processed prior to release into circulation, resulting in an ineffective hormone. Problems with the hormone receptor or the signaling pathway can also result in hormone resistance. Partial defects can be compensated for by an increased hormone concentration and have little clinical consequence, so hormone resistance may be unrecognized except on routine laboratory studies.
Abnormalities of hormone transport and metabolism
Under ordinary circumstances, abnormalities of hormone transport or metabolism do not result in endocrine pathology. Hormone production is controlled by the level of free hormone, and consequently can be adjusted up or down to maintain a steady level of free hormone. Therefore, abnormalities of hormone transport or metabolism most commonly cause deviation of laboratory parameters from normal but do not cause either hyper- or hypofunction. There are exceptions to this which will be discussed in later chapters. The important point is to recognize that unusual hormonal values do not necessarily imply functional pathology.
Feedback/Errata